Dr. Lingling Wu, Department of Earth and Environmental Sciences, University of Waterloo
Dr. Wu specializes in low-temperature geochemistry, geomicrobiology, and isotope geochemistry, combining fieldwork, laboratory experiments, and geochemical modeling, to understand how inorganic and biologically-mediated processes shape the geochemistry of the Earth's surface. The overall theme of her research is to quantify the biogeochemical cycling of elements, including carbon, phosphorus, nitrogen, base cations, and metals, in both modern and ancient environments. She focuses on chemical weathering reactions that control the chemistry of the Earth's hydrosphere and atmosphere, and produce the basic constituents of soils.
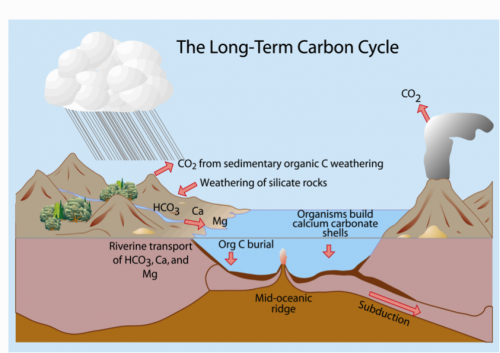
Figure 1 is a cartoon illustrating the long-term (greater than million-year) carbon cycle between atmosphere, hydrosphere, geosphere, and biosphere. Similar cycles also exist for other elements.
Of particular interest are microbe-rock interactions, which have likely contributed to the environmental evolution of Earth’s surface throughout geologic time. Since the origin of life, microbial metabolism has fundamentally altered geochemical cycles operating at the Earth’s surface. Microbes impact their environment, and in turn, the environment shapes microbial communities. Specifically, microorganisms influence the chemistry of aquatic systems either directly through the acquisition of energy released from redox reactions and/or limiting nutrients such as phosphorus, nitrogen required for biomass synthesis, or indirectly through the release of metabolites that lower pH, complex cations, and/or change mineral saturation states.
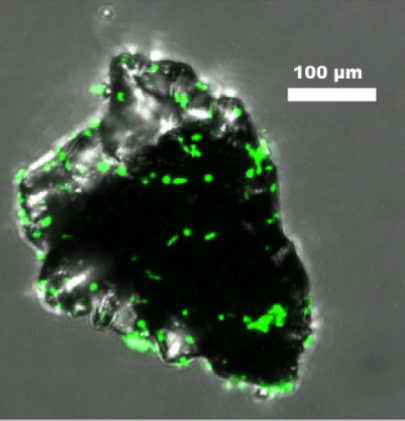
Figure 2 shows Burkholderia fungorum (a gram-negative, heterotrophic bacterium) colonizing a basalt particle.
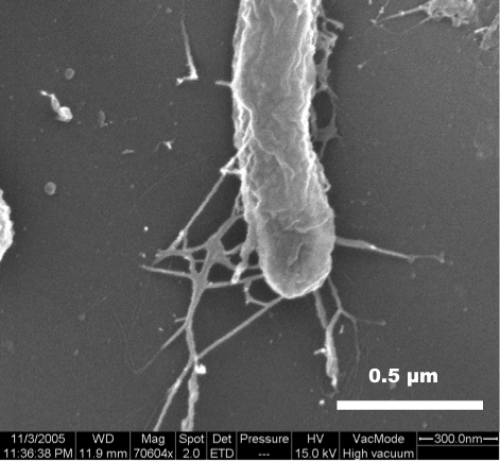
Figure 3 shows Burkholderia fungorum excretes EPS (extracellular polymeric substances) in order to stick on the surface of basalt.
Dr. Wu's current research focuses on investigating stable Fe isotope fractionations during interactions between Fe(II) and Fe-bearing phyllosilicates, in both biological and abiological experimental systems. The Fe-bearing phyllosilicates are wide-spread in subsurface environments and have been recognized as an important terminal electron acceptor by a group of microorganisms called dissimilatory iron reducing bacteria (DIRB). A solid understanding on stable Fe isotope fractionations during redox transformations of Fe(III) oxides has been achieved in the last decade, through both experimental and field work. As a contrast, much has yet to be learned during microbial reduction of Fe-bearing phyllosilicates. This project investigates the stable Fe isotope fractionations during interactions between ferrous iron and Fe-bearing clays, in both biological and abiological systems. The goal is to understand the mechanism and fractionation factor for isotopic exchange during these processes, which can then be applied to interpret Fe isotope data in natural systems. Field work will characterize Fe isotope signature in lake sediments, aiming at a better understanding of coupled Fe and P cycling.
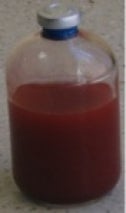
Figure 4 shows a sealed hematite (an iron(III) oxide) reactor containing iron-reducing bacteria Geobacter sulfurreducens. In order to prevent rapid oxidation of ferrous iron at neutral pH, all experiments have to be carried out in strictly anaerobic conditions.
Future effort will be devoted into probing nature and reactivity of surface phases during coupled Fe oxidation and Cr reduction using both Fe and Cr isotopes. Microbial dissimilatory iron reduction produces ferrous iron, which is a very efficient reducing agent in the presence of mineral surfaces. It can reduce Cr(VI), which is highly soluble, mobile and toxic, to insoluble Cr(III) that readily absorbes onto mineral surfaces and less toxic. A variety of Fe oxyhydroxide substrates including hematite, goethite, and poorly crystalline ferrihydrite will be examined to probe the nature and reactivity of surface phases formed during this coupled process using Fe isotope fractionations. As a second stage of this project, Cr isotope fractionations will be investigated, which system is largely underexplored compared with Fe isotope system. Other project will examine the impact of microbial N utilization from rocks on chemical weathering. This project quantifies how and to what extent microorganisms affect chemical weathering rates when they utilize N-bearing rocks. Minerals comprising sedimentary rocks, such as feldspars and clays, contain ammonium as a substitute for potassium. Traditional models of the N cycle emphasize nitrogen fixation from atmospheric N and uptake of N from soil organic matter but do not consider microbial utilization of N in rocks. This could have significant influence on chemical weathering rates and consequently the evolution of atmospheric CO2. This could shed new light on the terrestrial N cycle.