Classical Density Functional Theory
Dynamic Density Functional Theory (DDFT) builds upon Classical Density Functional Theory (cDFT) to model the time-dependent evolution of fluid densities in non-equilibrium systems. DDFT describes density dynamics using an integro-differential equation derived from the equilibrium Helmholtz free energy functional by generalizing Fick's law to include interacting particles. Beyond its roots in equilibrium cDFT, DDFT shares connections with phase-field crystal (PFC) models and power functional theory (PFT), broadening its scope to a wide range of non-equilibrium phenomena. It is particularly effective in capturing density variations down to the particle scale. DDFT finds applications in microstructure evolution, ionic fluid, and biological systems, providing critical insights into phase transitions, transport processes, and structural organization in complex materials.
Machine Learning
Machine learning is revolutionizing materials science with advanced models like diffusion, normalizing flow, and physics-informed models, each addressing critical challenges in material design and optimization. Diffusion models simulate material microstructures, normalizing flow models capture complex probabilistic relationships among properties, and physics-informed models integrate scientific laws to ensure physically consistent predictions. These tools enable high-constrained, multi-objective optimization, which is essential for accelerating novel materials' discovery and performance enhancement and driving innovation across the field.
Molten Salt Reactor
Achieving net-zero carbon emissions by 2050 is a significant challenge that will necessitate a substantial increase in clean energy sources, including nuclear power. Molten salt reactors (MSRs), which use salts as both fuel and coolant, offer potential advantages in efficiency and are particularly suited for non-electric applications. Originating from the Oak Ridge National Laboratory's 1950s Aircraft Reactor Experiment, MSRs were further developed during the Molten-Salt Reactor Experiment (MSRE) from 1965 to 1969, proving the concept of liquid-fueled reactors. With their exceptional heat absorption capacity, MSRs could operate at the high temperatures required for industrial processes like hydrogen production.
Diffusion in Solid
Diffusion is crucial in solid-state physics, metallurgy, and materials science, especially at elevated temperatures. For example, the absence of long-range atomic order in amorphous solids leads to unique diffusion behaviors critical for technologies like solid oxide electrolyzers and solid-state batteries. Understanding and controlling this process is essential to improving ionic conductivity, stability, and overall efficiency, driving advancements in energy storage and conversion systems.
Hydrogen Technologies
Hydrogen technologies play a pivotal role in the transition to a sustainable energy system, particularly in decarbonizing sectors that are challenging to electrify, such as shipping, steelmaking, and the chemical industry. Among the various methods for hydrogen production, Solid Oxide Electrolysis Cells (SOECs) stand out due to their exceptional efficiency and potential.
Unlike traditional water electrolysis, which is often limited by lower electrical efficiency, SOECs can achieve nearly 100% electrical efficiency. This remarkable performance is attributed to their favorable thermodynamics and kinetics, enabling higher hydrogen production rates. As renewable electricity prices continue to decline, SOECs are becoming increasingly vital for producing green hydrogen at scale.
Beyond production, the efficient storage of hydrogen is essential for its integration into the energy grid and various industrial applications. Advances in hydrogen storage technologies will be critical to ensuring that hydrogen can be reliably supplied where and when it is needed, making it a cornerstone of the sustainable energy landscape.
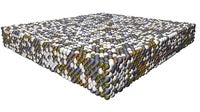
High-Entropy Alloys: A New Frontier in Material Science
High-entropy alloys (HEAs) are emerging as a groundbreaking class of materials with the potential to revolutionize various industries. Unlike traditional alloys, which are typically composed of one or two principal elements, HEAs consist of five or more elements mixed in near-equiatomic proportions. This unique composition results in remarkable properties, such as enhanced strength, corrosion resistance, and thermal stability, making HEAs ideal for applications in extreme environments like aerospace, energy, and defense. As research advances, HEAs are poised to become a cornerstone in the development of next-generation materials that meet the demands of modern engineering challenges