Prof. Linda F. Nazar
Department of Chemistry
University of Waterloo
200 University Avenue West
Waterloo, Ontario, N2L 3G1 Canada
Tel: (519) 888-4637
Fax: (519) 746-0435
Email: lfnazar@uwaterloo.ca
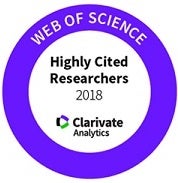
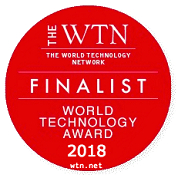
Research areas
Summaries of the Nazar Group's research interests are listed below. Click on the titles of the individual projects to jump to specific project descriptions.
Mg-Ion Batteries
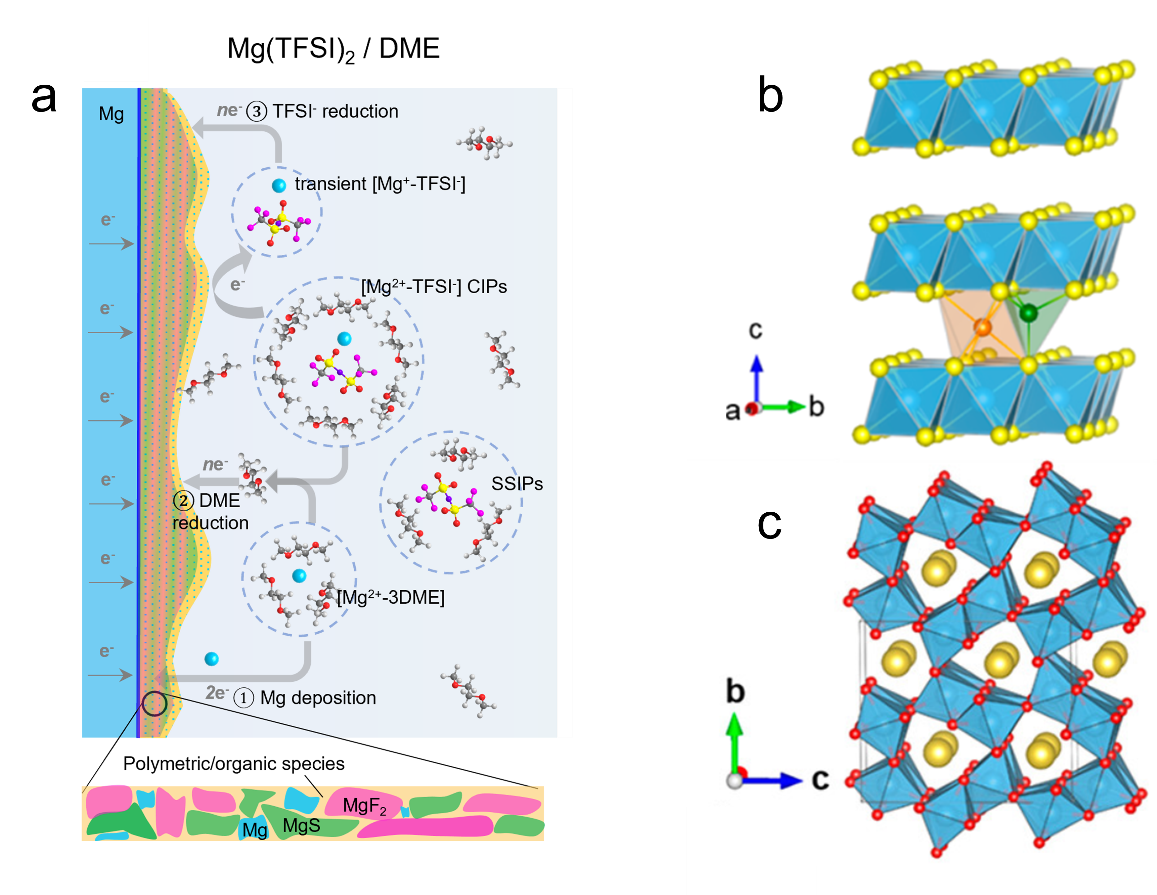
a) Scheme of interfacial reactions at Mg/electrolyte interface in convention Mg(TFSI)2/DME electrolyte. b) The crystal structures of layered TiS2 and c) tunnel-typed NaV1.25Ti0.75O4.
Rechargeable magnesium batteries (RMBs) stand out as a new battery technology due to the Earth’s abundance of Mg (over 1000 times that of Li),the high theoretical volumetric energy density of a Mg metal anode (3833 mAh cm-3 vs.2046 mAh cm-3 of Li), and magnesium’s relatively low reduction potential (-2.38 V versus a standard hydrogen electrode). However, the charge-dense Mg2+ cation results in: 1) a high desolvation penalty due to the slow kinetics of ion pair dissociation; 2) very slow ion migration kinetics because of a thick solid electrolyte interface on the Mg surface; and 3) sluggish ion migration in most inorganic intercalative-type cathodes.
In our group, we aim to develop highly efficient Mg-ion electrolytes that can tackle the interfacial dilemma at the Mg/electrolyte interface by tuning the electrolyte network and Mg2+ solvation structures to control the interfacial electrochemistry. At the cathode side, we help to develop the fundamental understandings of “soft” Mg2+-S bonds that can mitigate sluggish Mg2+ solid state diffusion. We are also screen high-voltage inorganic cathode materials for possible use in Li/Na cathodes.
Aqueous Batteries
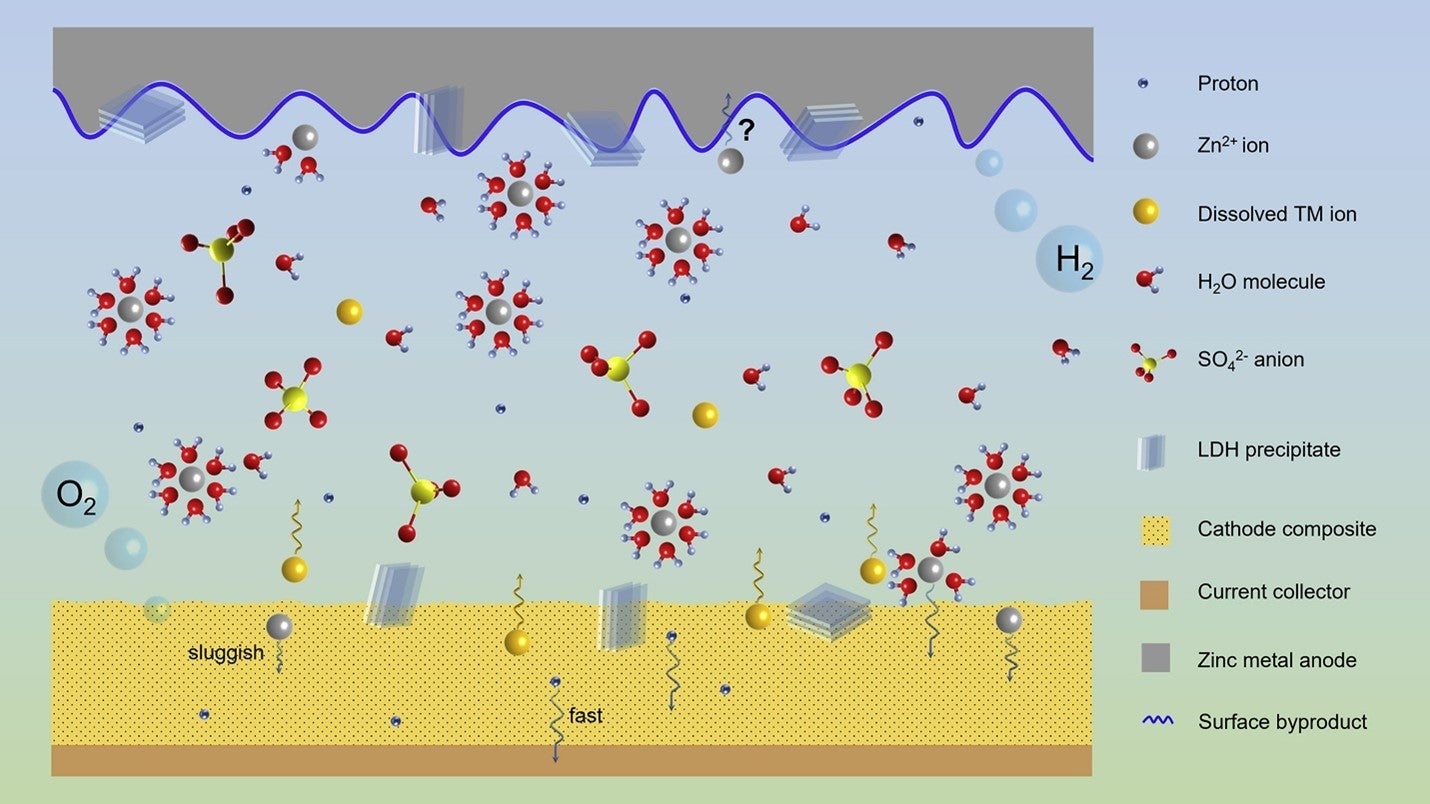
Schematic diagram of a prototypical aqueous ZMB [Li et al, Joule, 6 (8), 1733-1738, (2022)].
The scarcity of lithium resources and safety issues of lithium-ion batteries have diverted research attention to aqueous batteries due to their inflammability, easy manufacturing, and high ionic conductivity. Our lab focuses on aqueous Zn metal batteries (ZMBs), whose low cost and high safety make them highly promising for large-scale and stationary applications like grid energy storage.
Addressing the fundamental challenges that hinder the development of commercialized ZMBs has been the core of our research. Principle studies involve understanding the mechanism of failure modes such as Zn dendrites and side reactions like HER and OER, and finding cures accordingly. Our investigations primarily focus on studying and tuning aqueous electrolytes to achieve highly reversible and efficient Zn cell cycling and realizing new mechanisms and chemistries in ZMBs. The insights from these studies can be applied to the development of future aqueous batteries in general with multi-valent metal anodes other than Zn.
Solid-State Electrolytes (Sulfides and Halides)
All solid-state batteries (ASSBs) employing inorganic solid electrolytes offer improved safety and are exciting candidates for next-generation energy storage. A highly conductive SE with a wide electrochemical stability window is a key component to realize the promise of ASSBs. Superionic conductors can exhibit liquid-like and exceptionally high alkali cation conductivity in their crystalline lattices. We aim to achieve a fundamental understanding of the nature of superionic behaviour at the atomic level—the origin of the underlying high conductivity—to further increase ionic conductivity and eventually design new superionic conductors. We are broadly interested in a range of materials, including oxides, phosphates, sulfides, and halide ion conductors.
Solid-State Batteries and Interfaces
Developing solid-state batteries (SSBs) is one of the most promising research directions toward high-energy density batteries for EV applications. Compared to the conventional Li-ion batteries with organic carbonate electrolytes, SSBs exhibit much higher theoretical energy density and significantly reduced safety hazards.
Our group is focused on designing high voltage (>4 V) SSBs with an areal capacity above 3 mAh.cm-2 and a lifetime exceeding 500 cycles. Our SSBs employ high-capacity Ni-rich NCM cathode materials and advanced electrolytes with high (> 5 mS.cm-1) ionic conductivity and electrochemical stability above 4 V. Furthermore, we are developing SSBs with Li and Si anodes to maximize the battery energy density.
Since the long-term stability of SSBs is rooted in the interfacial stability between different cell components, we carefully explore the formation and evolution of various interfaces formed in the SSBs. We employ a variety of characterization techniques (EIS, ToF-SIMS, XPS) to study the behavior of interfaces during the cycling of the SSB. Our approach allows us to systematically investigate and boost the performance of the SSBs.
Na-Ion Batteries
Our group works on strategies to develop new sodium solid-ion conductors including inorganic synthesis and physical and structural characterization. We focus on developing the fundamental understanding of interfaces and ion/electron dynamics involving techniques such as XPS, ToF-SIMS, and micro–CT. We also work to elucidate the fundamental challenges of the hetero-ionic interfaces to understand the physicochemical processes that are crucial for all sodium solid-state batteries.
Lithium-Sulfur Batteries
Lithium-Sulfur (Li-S) batteries have received a lot of attention as a promising next-generation electrochemical energy storage system, due to their low cost, environmental friendliness, high specific capacity (1675 mAh g-1), and energy density (2600 Wh kg-1).
Our group began to research on Li-S batteries in the late 2000s using a promising cathode created by impregnating sulfur into a mesoporous CMK-3 carbon host. Many important discoveries in the Li-S battery field have been found by our group, including suppressing lithium dendrite growth, optimizing the composition of the electrolyte, and designing more efficient cathodes.
We also focus on all-solid-state Li-S batteries with high safety and no shuttle effect of polysulfide. To solve the challenges of all-solid-state Li-S batteries (slow ion/electron conductivity, solid-state electrolyte degradation, poor contact, unstable lithium/electrolyte interface), we have undertaken many efforts to design novel solid-state electrolytes, stabilize the lithium metal anode, and improve ion/electron conductivity of the cathodes.
Coatings to Stabilize High-Voltage Cathode Materials
Our group works to synthesize and fabricate new inorganic coating materials for high voltage cathodes used in solid state batteries. We also study the processes to apply these coating materials with controllable thickness and uniformity onto high voltage cathodes such as LNMO and NMC. The goal is to achieve stable cycling of full cell batteries utilizing these cathodes materials.
Computation
Our research group harnesses the immense potential of high-performance computing to delve into the intricate world of materials science. Equipped with the precision of modern electronic structure methods such as density functional theory, and guided by the fundamental principles of thermodynamics, our mission is to unravel the complex challenges that materials and interfaces face within various battery chemistries. Our ultimate goal is to elevate the energy density, power, reversibility, and lifespan of batteries to unprecedented levels.
Our research places a particular emphasis on the pursuit of innovative descriptors that can enhance the ionic diffusion within solid electrolytes. To achieve this, we employ a multifaceted approach that includes high-throughput density functional theory, molecular dynamics simulations, and state-of-the-art machine learning techniques. The descriptors we uncover are then utilized to design novel materials that are pivotal for the next generation of battery applications.