By: Michael C. Moncur
Introduction
Canada is one of the world's leading producers of economic minerals. The mining of base and precious metals results in the production of immense quantities of waste rock, mill tailings, and waste related to refining processes. The generation of acid mine drainage (AMD) and release of water containing elevated concentrations of metals from mine wastes is an environmental problem of global scale. AMD is caused by the oxidation of sulfide minerals in the mine waste that occurs when these materials are exposed to atmospheric oxygen (O2). This oxidation can continue to release acid and metals to the surrounding environment for decades to millennia (Moncur et al., 2005).
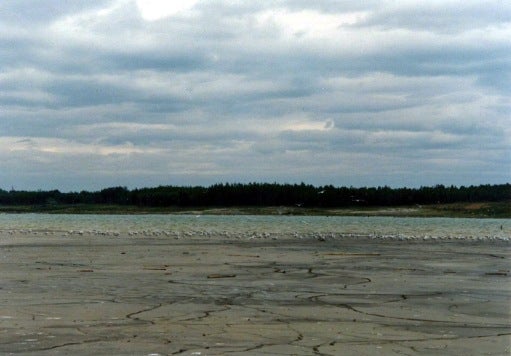
Above: Relatively fresh tailings in an impoundment.
Below: The same tailings impountment as Figure A (above) after 7 years of sulfide oxidation. The white spots in Figures A and B are gulls.
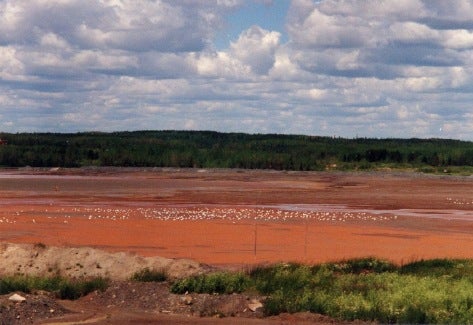
The annual worldwide production of mine wastes exceeded 4.5 giga-tonnes in 1982 (ICOLD, 1996). The annual production of mine wastes in Canada was 650 million tonnes in 1991, adding to the billions of tonnes of waste already accumulated (Government of Canada, 1991). In the United States it is estimated that more than 22 000 km of streams and 180 000 acres of freshwater reservoirs are adversely affected by AMD which will eventually cost US taxpayers between $32 billion and $72 billion to remediate (Kleinmann et al., 1991). In Canada, even though the mining industry spends over $100 million annually in the collection and treatment of mine effluent, estimates for the cleanup of existing AMD sites are between $2 and $5 billion (EMCBC, 2000). Estimated costs for remediating mine wastes internationally total in the tens of billions of dollars (Feasby et al., 1991).
The ratio of economic and precious metals recovered to mine waste produced is very low. For example, the average grades of copper deposits in Canada are less than 1%, meaning that for every tonne of copper produced 99 tonnes of waste material is generated (EMCBC, 2000). The wastes associated with gold mining provide additional examples on a scale most people will be familiar with. In order to extract enough gold for a typical wedding band about three tonnes of waste material will be generated (EMCBC, 2000).
History
There are documented accounts of environmental problems associated with mining that can be traced back thousands of years. As early as 2750 BP descriptions of AMD were made by the Phoenicians of Southern Spain when they discovered a river the colour of red wine flowing into the blue waters of the Mediterranean Sea. The source of the red water was one of the world's largest sulfide deposits now known as the Rio Tinto Mine. Even in Greek and Roman times concerns about the environment effects of mine drainage were noted (InfoMine, 2005). The German physician Agricola Georgius expressed his concerns over the environmental impacts of mining in his book De Re Metallica (1556) and stated, "The strongest argument of the detractors is that the fields are devastated by mining operations... Further, when the ores are washed, the water which has been used poisons the brooks and streams, and either destroys the fish or drives them away. Thus it is said, it is clear to all that there is greater detriment from mining than the value of the metals which the mining produces". While AMD is a more recent problem in North America, references to mine related impacts can be found as early as 1698 by G. Thomas. The long history of mining and mining wastes gives us some perspective for evaluating the potential longevity of the environmental impacts associated with mining activities. Recent studies of an ancient mining site in Southern Jordan have revealed elevated concentrations of metals in soils adjacent to the mine wastes despite 2000 years of weathering.
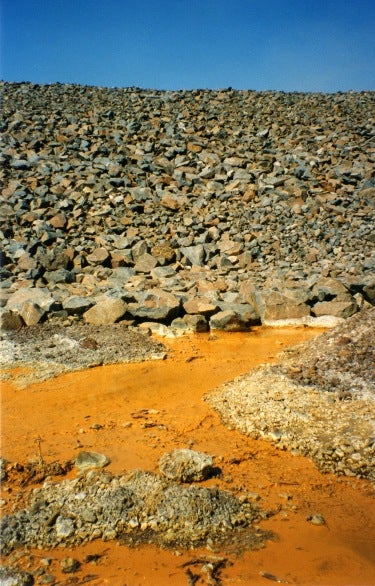
Above: Mine effluent discharging from the bottom of a waste rock pile.
Sulfide oxidation and the generation of AMD
The principal environmental concerns related to mine wastes are the oxidation of sulfide minerals within the waste materials and the subsequent release of oxidation products to the adjacent groundwater and surface waters. The most common sulfide minerals in mine wastes are pyrite [FeS2] and pyrrhotite [Fe1-xS] both of which have little commercial value. For example, the oxidation of pyrite by atmospheric O2 can by represented by:
(1) FeS2(s) + 7/2O2(g) + H20(1) à Fe2+(aq) + 2SO42-(aq) + 2H+(aq)
In reaction (1) when 1 mol of pyrite is oxidized, 1, 2 and 2 moles of Fe(II), SO4 and acid are produced, respectively. The Fe(II) generated by reaction (1) can be further oxidized to form Fe(III), which under mildly acidic to near-neutral pH conditions, will precipitate as a ferric oxy-hydroxide:
(2) Fe2+(aq) + 1/4O2(g) + 3/2H2O(1) à FeOOH(s) + 2H+(aq)
Under very low pH conditions (pH<3), Fe(III) can remain in solution and react with the sulfide minerals through:
(3) FeS2(s) + 14Fe3+(aq) + 8H20(1) à 15Fe2+(aq) + 2SO42-(aq) + 16H+(aq)
Reaction (3) indicates that for every mole of FeS2 oxidized by Fe(III), 16 moles of acid are generated. In addition to the oxidation of pyrite and pyrrhotite, the oxidation of other sulfide minerals that may be associated with the mine wastes, including chalcopyrite [CuFeS2], sphalerite [ZnS], galena [PbS], and arsenopyrite [FeAsS] may also occur. The acidity released from the sulfide oxidation reactions is of concern because the low-pH solutions increase the solubility of potentially toxic trace metals and semimetals such as Pb, Zn, Cu, Cd, Cr, Co, Ni, Al, Sb, and As, making them more mobile. In dissolved form, metals are more readily absorbed and accumulated by plant and animal life and therefore more toxic than they are in solid phase (EMCBC, 2000). The uptake and accumulation of metal by aquatic life can be passed along the food chain and may also damage subaqueous and subareial habitats. In trace amounts, metals are essential for life, however, in high concentration they can be very toxic.
Below: Shoreline of a pond receiving AMD showing massive accumulation of iron hydroxides on the pond bottom.
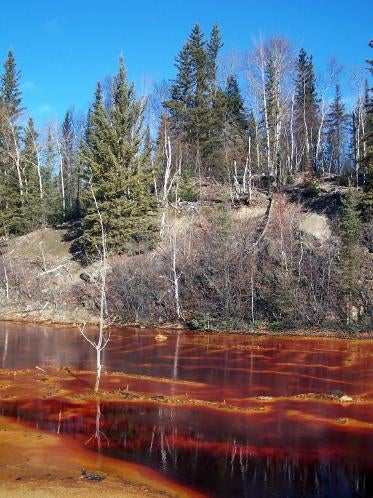
Acid neutralization reactions
The acid produced through the oxidation of sulfide minerals reactions can be neutralized along the groundwater flow path through reaction with gangue minerals incorporated in the mine wastes. Example gangue minerals which can contribute to acid neutralizing reactions include carbonate minerals and aluminosilicate mineral phases. Dissolution of these gangue minerals typically leads to a distinct sequence of pH-buffering plateaus (Blowes et al., 2003). The final composition of pore waters in a mine waste pile will depend on the composition and abundances of the sulfide minerals and the composition and abundances of the gangue minerals. The ratios of the various phases and reaction rates will determine whether a waste pile will be acid generating or remain neutral over time.
Ore and country rock typically contain carbonate minerals such as calcite [CaCO3], dolomite [CaMg(CO3)2] and ankerite [Ca(Fe,Mg, Mn)(CO3)2]. In the early stages of sulfide oxidation, the dissolution of carbonate minerals, such as calcite, results in maintaining the pH of the pore water near neutral (pH 6.5-7.5) through:
(4) CaCO3(s) + H+(aq) <--> Ca2+(aq) + HCO3(aq)
In reaction (4) the dissolution of one mole of calcite results in the consumption of one mole of acid. The pH of the pore water will remain neutral providing that there is a sufficient mass of primary carbonate minerals remaining in the mine wastes (tailings and waste rock). As calcite is depleted the pore water pH decreases until a mineral with a lower solubility, siderite [FeCO3], forms the next pH buffering plateau:
(5) FeCO3(s) + H+(aq) <--> Fe2+(aq) + HCO3(aq)
The dissolution of siderite will buffer the pore water between a pH of 4.8 to 6.3. As the dissolution of carbonate minerals occur, the dissolution of the most soluble aluminosilicate minerals (eg. biotite [K(Fe)3[AlSi3O10(OH)2]) may release dissolved aluminium to the pore water and precipitate as gibbsite [Al(OH)3] or another Al hydroxide mineral phase:
(6) Al(OH)3(s) + 3H+(aq) <--> Al3 + 3H2O(s)
When siderite has become depleted, the dissolution of gibbsite will maintain the pore water pH between 4.0 to 4.3. Upon the dissolution of of the Al hydroxide phase, the dissolution of Fe oxyhydroxides will be the primary buffering mineral maintaining the pore water pH between 2.5 and 3.5:
(7) FeOOH(s) + 3H+(aq) <--> Fe3+(aq) + H2O(l)
After the depletion of the carbonate minerals, soluble Al hydroxides and Fe oxyhydroxides has occurred, the dissolution of aluminosilicates becomes the primary process contributing to acid neutralization.
Tailings impoundments
Mine tailings are the fine-grained material (clay sized to medium sand) that remains after the ore extraction process. The surface area of tailings impoundments can range in area from a few ha to several km and from 2 to 50 m in depth. The tailings are typically deposited into an impoundment as a slurry with up to 30 wt.% solids. After the tailings disposal is complete, the water table within the impoundment falls to an equilibrium position controlled by the rate of precipitation, evapotransperation, and hydraulic properties of the tailings and underlying geologic materials (Blowes et al., 2003). The drainage of the tailings enhances their structural stability but also leads to sulfide oxidation within the unsaturated zone (vadose zone).
Below: Groundwater flow through a tailings impoundment and discharging into lakes or streams.
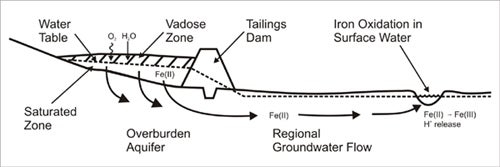
The atmospheric O2 which diffuses into the vadose zone reacts with the sulfide minerals within the tailings, releasing acid, Fe2+ and metals to the adjacent pore waters. Metal laden water affected by the sulfide oxidation reactions in the tailings is gradually displaced downward through the impoundment into the underlying geological materials by the infiltration of rain water. Ferrous iron is relatively soluble and the water flowing through the tailings commonly contains elevated concentrations of Fe2+ (Blowes and Cherry, 1991). The Fe2+-rich groundwater may travel along groundwater flow paths for years to decades before discharging to lakes or streams. This change from reducing to oxidizing conditions at the freshwater interface will result in the oxidation of Fe2+ and the subsequent precipitation as an oxy-hydroxide (equation 2) generating acidic conditions which could have large impacts on aquatic life.
Waste rock piles
The term waste rock is used to describe the coarse grained component of mine waste and includes rock that cannot be processed economically ranging in size from fine-grain sands to blocks several meters in diameter. Waste rock piles range in height from 30-50 m and can cover several kilometres in area (Blowes et al., 2003). As with mine tailings the oxidation of sulfide and subsequent generation of acid is the greatest environmental concern associated with waste rock piles, however, there are a couple of key differences between the two types of waste. Due to the coarse nature of the waste rock, the rock piles tend to have very high permeabilities allowing the penetration of large volumes of atmospheric O2 which promotes sulfide oxidation. In addition to increased penetration of O2 because of high permeabilities, very high temperatures within waste rock piles (e.g. in excess of 60oC) can further induce oxidation reactions. Waste rock piles were often constructed on permeable geologic material; acid and metals released to the pore water can be displaced downwards into the underlying geologic material by infiltrating precipitation.
Below: Precipitation of the secondary Fe mineral melanterite at a groundwater discharge zone in a tailings impoundment.
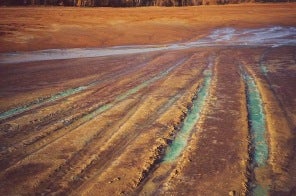
Remediation and prevention
There are a number of approaches to prevent and treat the degradation of groundwater and surface water from mining effluent. Among the various remediation strategies to treat AMD are the emplacement of covers to prevent the ingress of atmospheric O2 and infiltration of precipitation, collection and treatment of the contaminated groundwater and surface water, passive treatment of surface water using constructed wetlands, and permeable reactive barriers to treat the groundwater in-situ.
Immediately after the tailings or waste rock has been deposited, the first approach is to control the entry of atmospheric O2 and infiltrating water. This approach involves the addition of a cover over the mine wastes. Physical barriers that have been applied or proposed include water covers, soil covers of fine-grain material to maintain high moisture content, covers composed of organic materials, or the emplacement of a synthetic cover such as plastic, asphalt, or concrete. Covers also contain the tailings and waste rock preventing erosion by wind and water. To be most effective, covers must be applied either at the time that mine-waste deposition ceases or shortly thereafter because the rate of sulfide oxidation is greatest immediately after the mine waste is deposited (Blowes et al., 2003).
Collection and treatment involves collecting the mine effluent and treating the contaminated water prior to discharge from the mine site. This method of remediation is common and used at many mine sites in North America. For this method of treatment, the pH is neutralized through the addition of lime, with the subsequent precipitation of metal as a ferric hydroxide sludge. However, the cost of maintenance of these facilities is large and the disposal of the metal laden sludge produced from the treatment is an environmental concern.
Constructed wetlands perform as a passive water treatment facility. Contaminated mine effluent is directed into the wetland where metals are scavenged and the pH is increased through a combination of bioaccumulation reactions and sulfate reduction. This method of remediation has demonstrated to be efficient and effective but it is not known how long the wetland will remain stable over long periods of time or if the wetland will withstand the metal loadings.
Permeable reactive barriers are used to treat and prevent contaminated groundwater from mine sites that has infiltrated into underlying or adjacent aquifers. A trench is excavated through a portion of the aquifer down gradient from the mine disposal area. The trench is then filled with a permeable material composed of reactive components such as composted municipal wastes, wood wastes, by-products from pulp-and-paper manufacturing, zero valent iron, limestone, and phosphate based absorbent materials (Blowes et al., 2003). The reactive mixtures are designed to promote the bacterially mediated reduction of sulfate and precipitation of metals. Permeable reactive barriers have advantages over the conventional approaches for groundwater remediation because succeeding installation, the barrier requires little or no maintenance and its performance can persist for several years to decades.
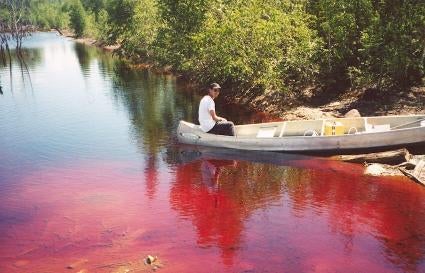
Above: Heading out for water sampling at an acid mine lake.
Conclusions
Although metals are essential for life and our current standard of living, the waste produced from the extraction of the metals may contain an abundance of sulfide minerals. The oxidation of sulfide minerals from the exposure to atmospheric O2 results in the generation and release of acid, Fe(II) and potentially toxic metals. Oxidation products transported from the mine site can discharge to streams and lakes having a negative impact on the water quality. Over the past few decades advancements in remediation technologies to prevent AMD are being applied to past and present mining operations thereby decreasing the impact to the surrounding environment. The next time you decide to dispose of an aluminium can, scrap iron, copper tubing, old car battery, or any other metal in the garbage instead of the appropriate recycling depository, think of the impact you my be causing not only to the environment but also to the economy.
References
Agricola, G. 1556. De Re Metallica. (tr. by Herbert Clark Hoover and Lou Henry Hoover) Dover Pub. Inc., 1950. New York. p. 638.
Blowes, D. Cherry, J. 1991. Mill tailings impoundments: geochemistry and hydrology. Hazardous Materials Managements. Vol. 3(3). pp. 6-11.
Blowes, D.W., Ptacek, C.J., Jambor, J.L., Weisener, C.G. 2003. The geochemistry of acid mine drainage. In: Environmental Geochemistry (ed. B.S. Lollar) Vol. 9 Treatise on Geochemistry (eds. H.D. Holland, K.K. Turekian). Elsevier-Pergamon, Oxford. pp. 149-204.
Environmental Mining Council of BC. 2000. Acid Mine Drainage: Mining and Water Pollution Issues in BC. Report available at: http://www.miningwatch.ca/.
Feasby, D.G., Blanchette, M., Trembly, G. 1991. The mine environment neutral drainage program. In 2nd Int. Conf. Abatment of Acid Drainage. MEND Secretariat, Tome. Vol. 1. p. 1-26.
Government of Canada. 1991. State of Canada's Environment. Ministry of Supplies and Services, Ottawa.
ICOLD. 1996. A Guide to Tailings Dams and Impoundments: Design, Construction, Use and Rehabilitation. International Commission on Large Dams, Bulletin (United Nations Evironmental Programme). No. 106. pp. 239.
InfoMine. 2005. Web page accessible at: http://www.infomine.com/.
Kleinmann, R.P.L., Edenborn, H.M., Hedin, R.S. 1991. Biological treatment of mine water - an overview. In 2nd Int. Conf. Abatment of Acid Drainage. MEND Secretariat, Tome. Vol. 1. p. 27-42.
Moncur, M.C., Ptacek, C.J., Blowes, D.W., Jambor, J.L. 2005. Release, transport and attenuation of metals from an old tailings impoundment. Applied Geochemistry. Vol. 20. p. 639-659.
Acknowledgements
Special thanks to Jean Birks and Carol Ptacek for reviewing and providing constructive comments and Peter Russell for his editing and assembling this article. I had the privilage of conducting the research for this article during my MSc. thesis under the guidance of Carol Ptacek and David Blowes.