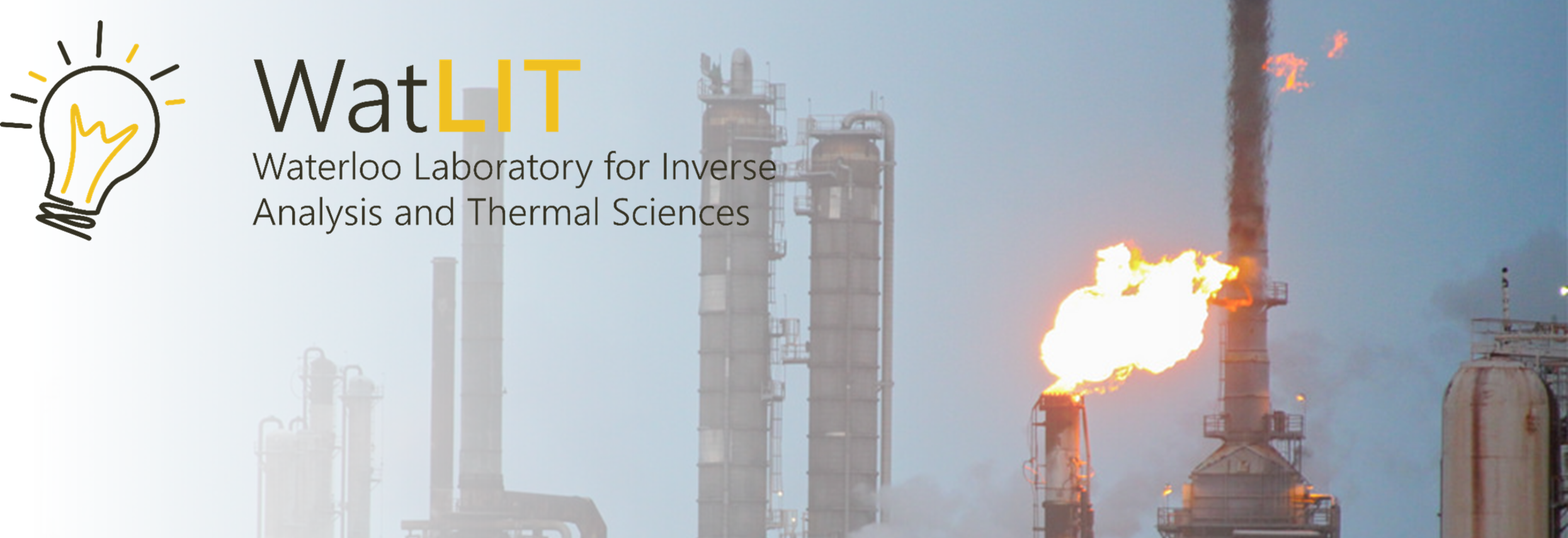
Why is this important?
Methane amounts to 30% of our greenhouse gas inventory; in Canada the majority of anthropogenic methane emissions come from the upstream oil and gas sector. The Government of Canada is committed to reducing these emissions to 40%-45% of 2012 levels as part of its Paris Accord commitments by 2035, through environmental regulation and industrial incentive programs. In order to do this, engineers, scientists, and policy makers need instrumentation that can accurately quantify methane emissions from various sources.
While methane is transparent over visible wavelengths, it absorbs and emits radiation at infrared wavelengths. Mid-wavelength infrared (MWIR) cameras are widely used by the oil and gas industry to visualize leaks during leak detection and repair surveys. However, there is an emerging trend towards using these cameras to quantify methane emissions: this is called quantitative optical gas imaging (QOGI).
How does QOGI work?
The brightness of each pixel in a MWIR image is proportional to the amount of infrared radiation incident on the camera along the corresponding line-of-sight (LOS) through the plume. The intensity is due to transmission of background intensity through the plume, and emission of intensity by the plume due to its temperature, and is modeled using the radiative transfer equation. Inferring the gas concentration along each LOS is mathematically ill-posed, so prior information (e.g. plume and background temperature) must be specified.
Concentrations are then combined with velocities that may be estimated from the apparent motion of pixels between successive images (optical flow) or from local anemometry data to obtain mass fluxes.
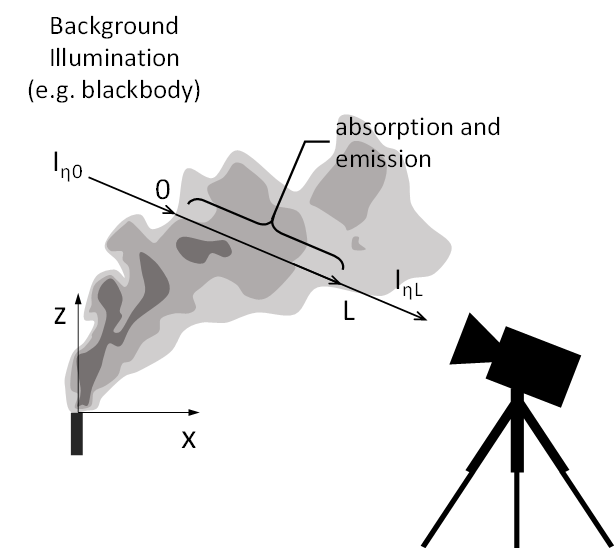
Multispectral and Hyperspectral Cameras
Most conventional QOGI measurements rely on a single filtered MWIR image. Unfortunately, uncertainly in the gas and background intensities, and the presence of other gases along the LOS, limit the reliability of QOGI methane flux estimates. More reliable estimates may be obtained using multispectral (MS) or hyperspectral (HS) cameras.
Multispectral cameras generate simultaneous or near-simultaneous MWIR images using filters with transparent windows over different parts of the spectrum. In principle it is possible to simultaneously infer both the gas concentration, gas temperature, and background temperature, by comparing the images recorded at different wavelengths. A major challenge with MS cameras concerns discerning radiation incident along the LOS with emission from the opaque portion of the filter.
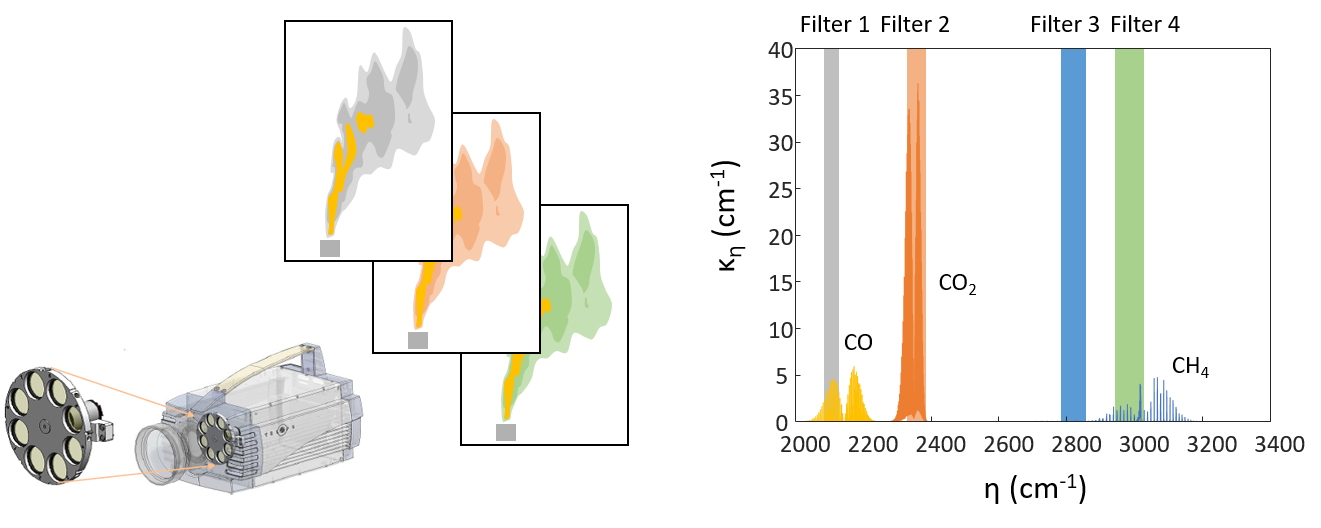
Hyperspectral cameras incorporate an interferometer to produce a “hypercube” of thousands of 2D images, each generated at a distinct wavelength. These cameras provide very accurate estimates of gas concentration and temperature, and can also be used to fingerprint unknown gases and gas mixtures.
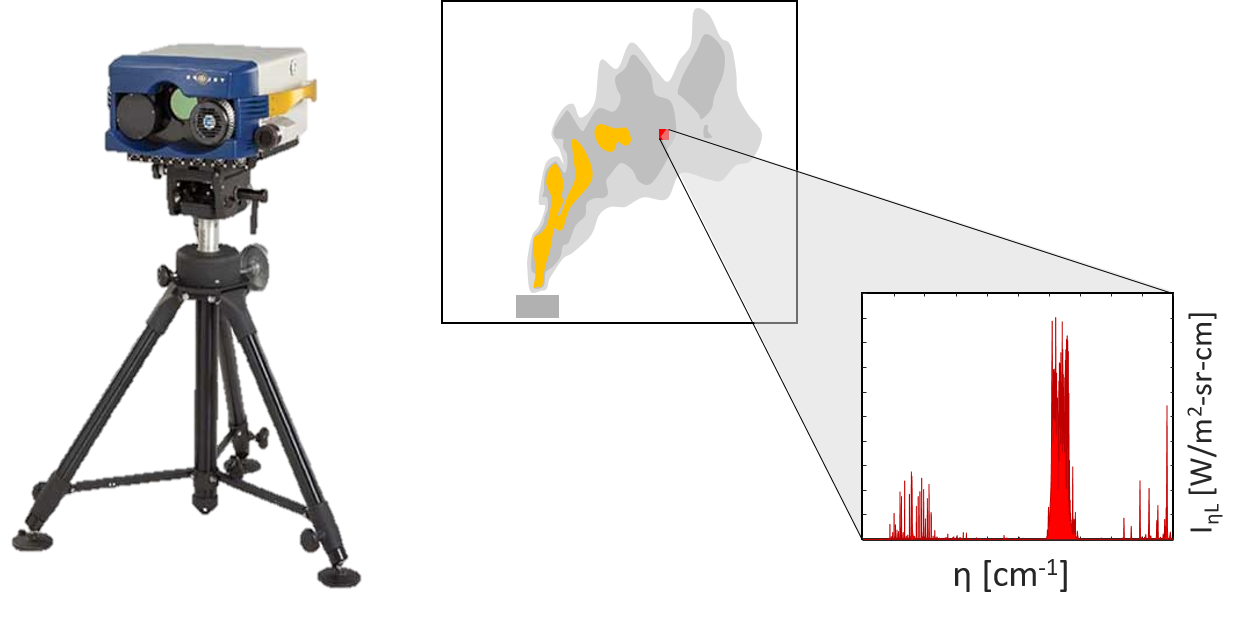
Experimental QOGI research
Our research into MS and HS cameras is focused on: (1) developing improved QOGI techniques for quantifying methane emission from upstream oil and gas facilities and (2) measuring the combustion efficiency of oil and gas flares.
The WatLIT lab has developed a unique apparatus for benchmarking the performance of MWIR cameras and QOGI algorithms. The apparatus consists of mass flow controllers that can produce a defined mixture and flowrate of up to four individual component gases. The gases flow through a heated line and discharge in front of a background of heated or cooled plates. Gas composition, temperature, and pressure can be chosen to simulate industrially-relevant scenarios.
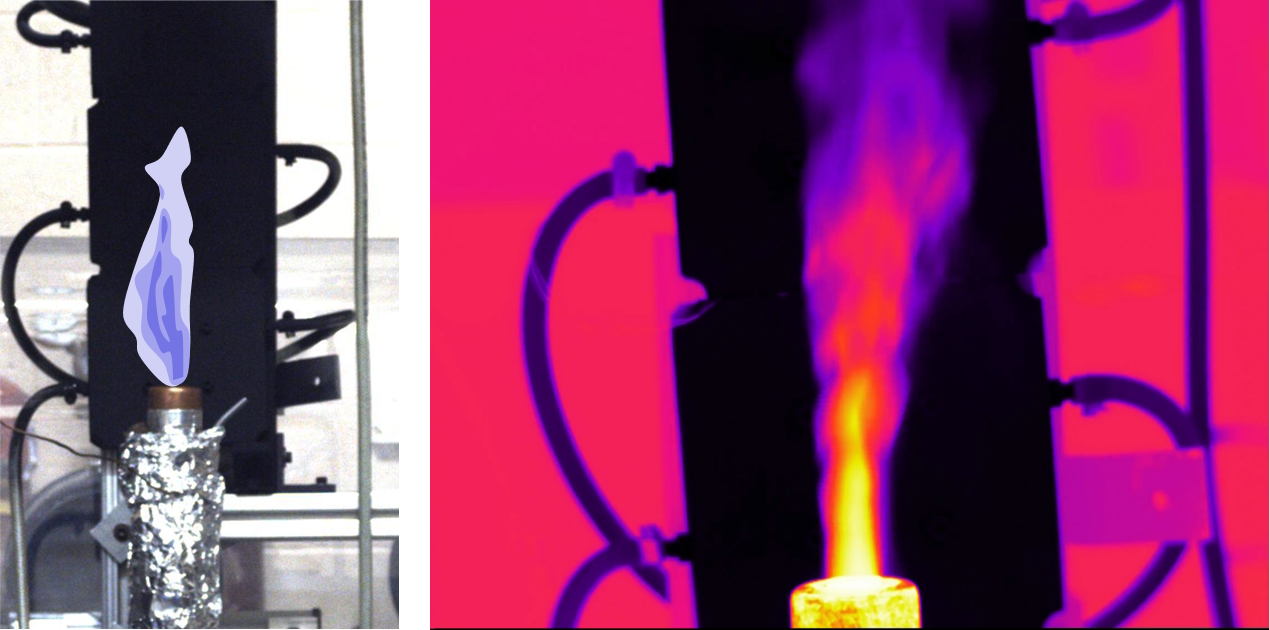
Flare combustion efficiency
Flares convert natural gas and other hydrocarbon byproducts of oil and gas extraction into CO2, which is safer and has a significantly lower impact on climate change. Under ideal operating conditions flares convert almost all the fuel into CO2, but cross-winds as well as air- and steam-assisted smoke suppression can significantly reduce flare combustion efficiency.
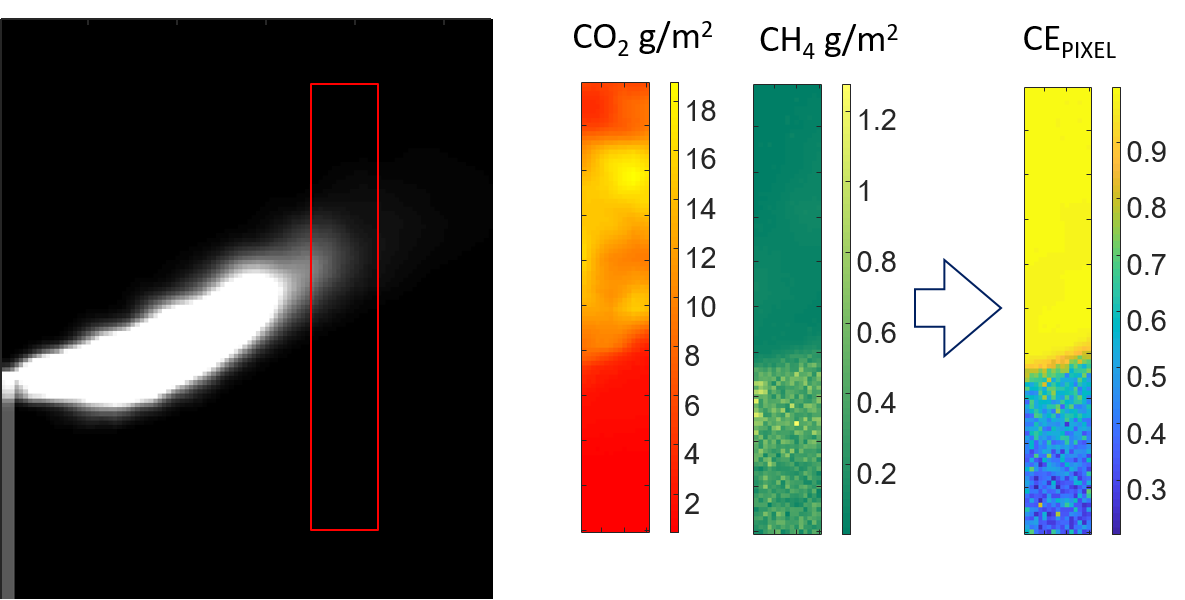
Hyperspectral imaging may potentially be used to quantify CO2 and CH4 column densities. The hyperspectral image below shows the plume of a lab-scale flare operating with steam assist. The intensity spectrum from a pixel outside of the plume can be used to calculate the ambient CO2 concentration and temperature and background temperature. This spectrum is compared with the spectrum from a pixel within the plume to identify and quantify column densities of CO2, CH4, and H2O within the plume.
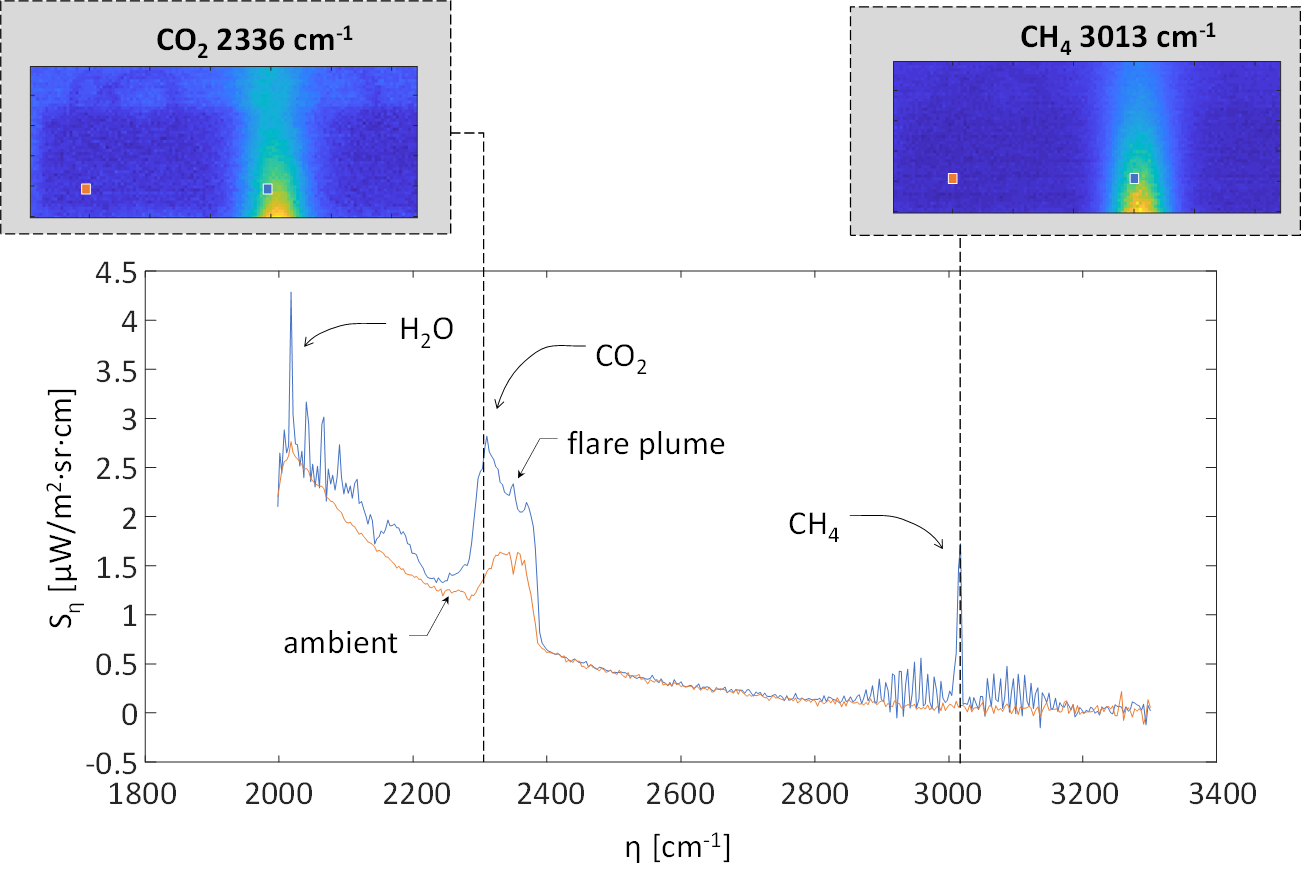
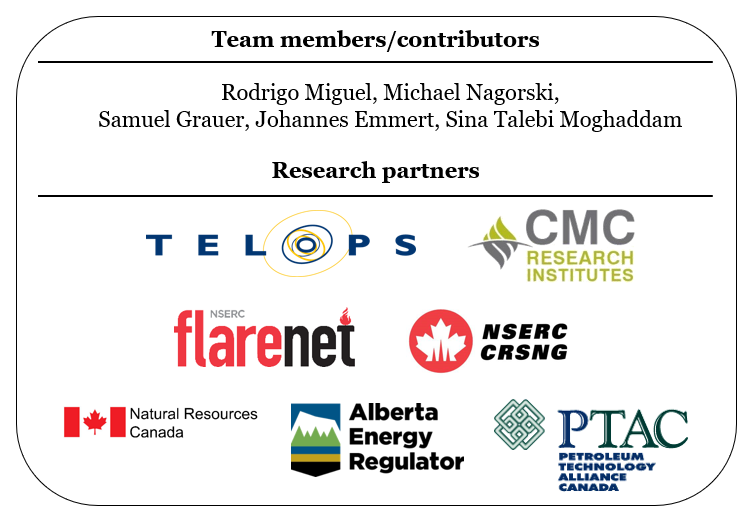