Why study microbial communities?
In the environment, microbes live in diverse communities, often with hundreds or thousands of different species. These organisms develop complex networks of interactions, including metabolic hand-off points within nutrient cycles, production and scavenging of co-factors, syntrophy, predation, and competition. The vast majority of environmental microorganisms have proven difficult or impossible to culture, in part because these interactions and networks are not maintained during isolation attempts. Environmental surveys and community-based approaches are currently the best way to characterize the diversity of microbial life on earth, and to identify novel metabolisms impacting global geochemical cycles or contaminants’ fates.
How to study microbial communities?
The Hug lab uses two main approaches to examine microbial communities. We sequence total community DNA (metagenomics), RNA (metatranscriptomics), and proteins (metaproteomics) from environmental samples. The DNA sequencing provides a blueprint of the microbial community, identifying the organisms present and allowing us to model the community’s metabolic potential. We take this one step further with genome-resolved metagenomics, where we can connect a given organism with specific predicted functions, through curation of draft genomes from metagenomes. The RNA and protein data allow us to identify what organisms are active in the environment and which processes are most abundant.
The second approach we use to study microbial communities is in the lab, with enrichment cultures and microcosms. These culture techniques aim to maintain a mixed population of bacteria and archaea. These methods reduce the total complexity of the community but maintain activities of interest, creating a system that is tractable for experiments.
Why study environments impacted by human activities?
There are no environments on earth untouched by human activities, between the impacts of climate change and global pollution. Understanding microbial adaptations and activities in contaminated or changing environments is critical for determining best-practices for waste management, agriculture, and contaminant remediation. Contaminated sites are often under-studied environments harboring previously unknown microbial lineages and metabolisms. An organism that evolved to respire, metabolize, or transform a contaminant in an impacted environment is often the most effective and efficient option for developing bioremediation tools.
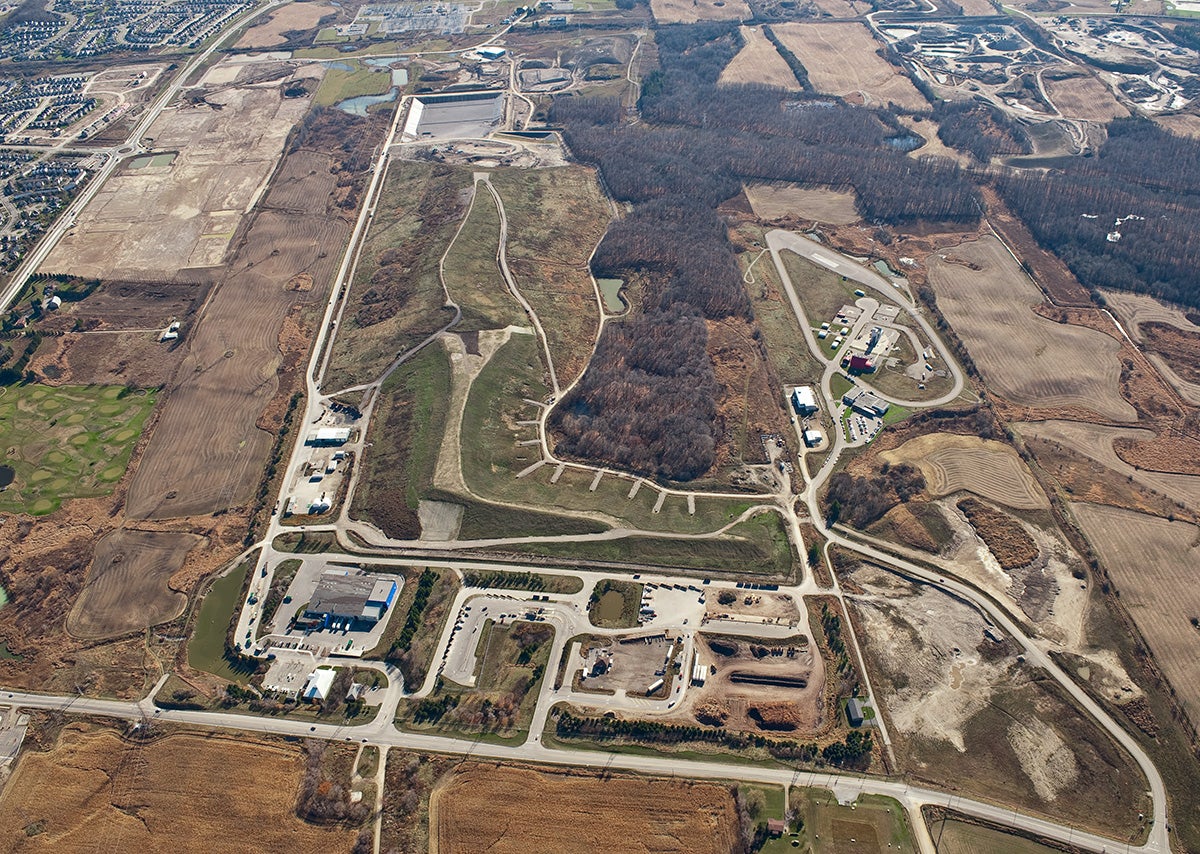
One of our current systems of interest: municipal landfills. Pictured: southern Ontario landfill, aerial view.
We have active collaborations with municipal landfills in Southern Ontario, Delaware, and California. Sampling the liquid leachate from these sites for meta-omics analyses and enrichment culture experiments, we identify and characterize the microbial populations. We are examining microbial community heterogeneity, phylogenetic diversity, predicted roles in geochemical cycles, and potential for in situ contaminant attenuation. Active projects include determining the potential for degradation of the common contaminant 1,4-dioxane as well as the emerging waste stream of bioplastics; the dynamics of methane cycling; prevention of biofilm formation within landfill infrastructure; and the impact of viral infections on the microbial community and ecosytem services.
We are examining metals cycling at Canadian mine waste sites, in order to understand microbial roles in metal contaminant mobility and the potential for extraction of rare earth metals from existing waste stockpiles.
We are involved in a research program examining soil microbial community responses to warming winters, which lead to greater frequency and intensity of freeze-thaw cycles and colder soils through the winter season. Our work examines the impact of increased freeze-thaw cycling on fertilizer availability, providing guidance to the agricultural community as to the optimal timing of fertilizer amendments.