Research Focus
Our lab conducts cutting edge research on advanced materials, which are suitable for electronics and electrical devices. Currently we are focusing on the development of printable electronic materials including polymer semiconductors, polymer conductors, polymer binders, and various nanomaterials for thin film transistors (flexible displays, RFID tags, smart labels, sensors, and wearable electronics), photovoltaics (solar cells and photodetectors), and batteries (lithium batteries).


Latest Published Article Related to Chemical Sensors
A novel donor–acceptor (D-A) conjugated polymer, poly[bis(2-ethylhexyl)3-(3′′,4′-bis(dodecyloxy)-[2,2′:5′,2′′-terthiophen]-5-yl)-1,4-dioxo-6-(thiophen-2-yl)pyrrolo[3,4-c]pyrrole-2,5(1H,4H)-dicarboxylate] (PDEB), comprising an electron-accepting carbamate side-chain bearing thiophene-flanked diketopyrrolopyrrole (DPPTh) and an electron-donating 3,3′-bis(dodecyloxy)-2,2′-bithiophene (C12-BTO) is synthesized. The carbamate side-chains in this polymer can be thermally removed at a moderate temperature, forming an insoluble polymer poly[3-(3″,4′-bis(dodecyloxy)-[2,2′:5′,2″-terthiophen]-5-yl)-6-(thiophen-2-yl)-2,5-dihydropyrrolo[3,4-c]pyrrole-1,4-dione] (PDNB) with high solvent resistance. PDNB has a high highest occupied molecular orbital energy level of −4.68 eV and a narrow band gap of 1.05 eV, which makes it dopable by HCl to form a stable conductive polymer PDNB:HCl with moderate conductivity of 0.24 S cm–1. A chemiresistive sensor based on PDNB:HCl can distinguish ten common volatile organic liquids with unique current–time profiles under a low operation voltage of 1 V. Importantly, a single sensor can be used many times and remain functional over a long period of time in air, indicating its extraordinary operational and environmental stability. The results demonstrate that PDNB is a promising material for reusable chemiresistive sensors.
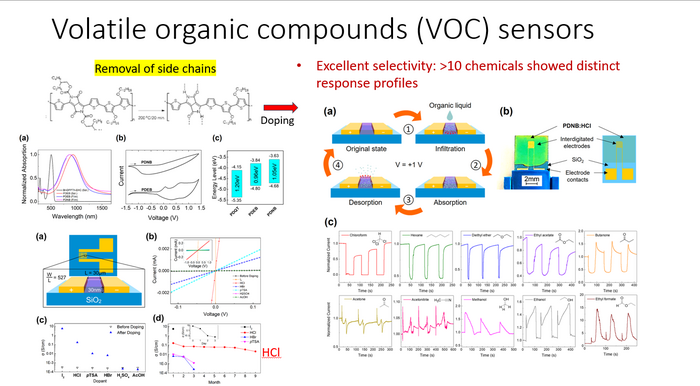
Latest Published Article Related to Rechargeable Battery Development
Lithium–sulfur (Li–S) batteries represent a promising high-density energy storage technology. The use of conductive polymers to enhance the performance of Li–S batteries has received much attention. In this work, a convenient and low-cost in-cell electropolymerization method is developed for the preparation of a conductive polymer, poly(3,4-ethylenedioxythiophene) (PEDOT), as a cathode binder inside a Li–S battery. The cell with this electropolymerized PEDOT (ePEDOT) shows notably improved specific capacity, cycling stability, and rate performance in comparison with the cell using the chemically synthesized commercial PEDOT:PSS (cPEDOT:PSS) as the binder. The performance enhancement is attributed to the formation of tightly integrated interfaces between ePEDOT and other components in the sulfur cathode. Moreover, this study revealed that the electrochemical dedoping of PEDOT is likely to occur during the Li–S battery cycling and thus the contribution of PEDOT (and some other conductive polymers) to the enhancement of the electrical conductivity of the sulfur cathode may not be as significant as expected.
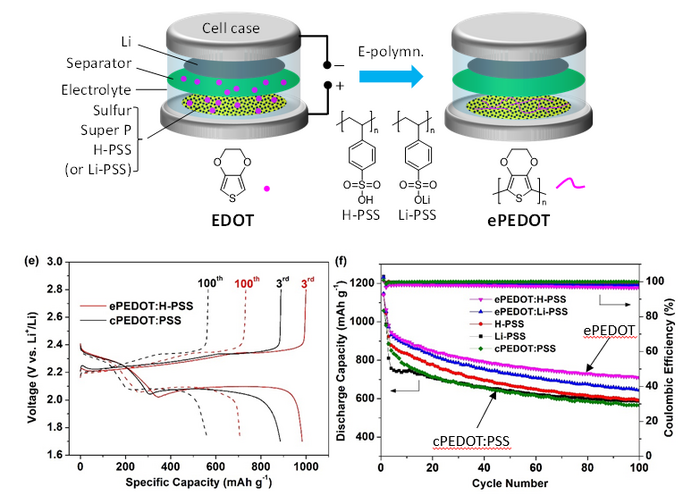
Latest Published Article Related to Organic Photovoltaics:
We studied the vertical and lateral charge transport characteristics of a diketopyrrolopyrrole polymer donor (D)–PC61BM acceptor (A) system by measuring the space charge limited current (SCLC) mobility and field-effect mobility respectively. It was found that with an increase in annealing temperature, the SCLC hole mobility decreased for the pure polymer (PDBFBT) but increased for the PDBFBT:PC61BM blends, which could be explained by changes in the crystallinity and crystal orientation (edge-on versus face-on). The pure PDBFBT and most blend films showed the maximum field-effect hole mobility (µh) when annealed at 100°C, which then declined as the annealing temperature was further increased. Surprisingly, the D/A = 1/1 blend films annealed at high temperatures exhibited an abrupt increase in the field-effect µh. This unusual phenomenon was interpreted by the antiplasticization effect of PC61BM, which promoted the molecular organization of the polymer. The effect of annealing on the carrier mobility was further correlated with the performance of inverted organic solar cell devices with the PDBFBT:PC61BM blend (D/A = 1/3). Thermal annealing at high temperatures (>100°C) was found to obstruct electron transport and cause the device performance to significantly deteriorate.
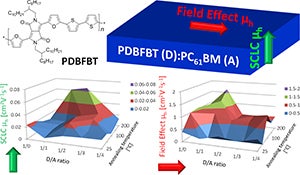