Contributors: L. Gui, R. Samy, T. Glawdel, C. L. Ren
On-chip temperature measurement is key to microfluidic chip applications involving heat transfer and becomes more prominent for polymer-made chips due to their low thermal conductivities. Many methods have been developed for on-chip temperature measurement among which the method involving the mixing of a temperature-dependent fluorescent dye (Rhodamine B) with working liquid [Figure 1], is very promising. However, it is difficult to apply this method to polymer-made chips because fluorescent dye particles can quickly diffuse into polymer channel walls causing significant measurement errors, as shown in Figure 1 below.
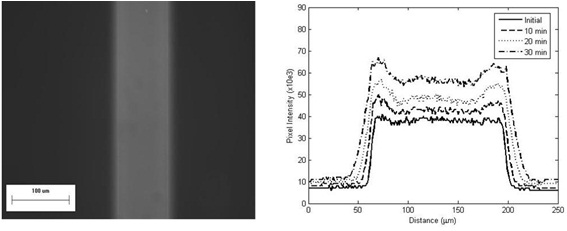
Image of a 100μM Rhodamine B filled polydimethylsiloxane (PDMS) microchannel after 30 minutes (left) and the corresponding fluorescent intensity profile at 10, 20 and 30 minutes. Absorption and adsorption is evident due to the detectable fluorescent intensity extending beyond the channel walls, and the peak intensities occurring at the solid-liquid interface.
There have been three methods (Method I, Method II and Method III) developed in the Waterloo Microfluidics Laboratory to resolve this problem while realizing on-chip temperature measurements. The first two methods involve the use of a PDMS film saturated with Rhodamine B dye which is bonded with the working chip, while the third method focuses on improving the temperature measurement accuracy by removing the fluorescence of absorbed and adsorbed Rhodamine B dye particles in the surrounding chip material.
Method I:
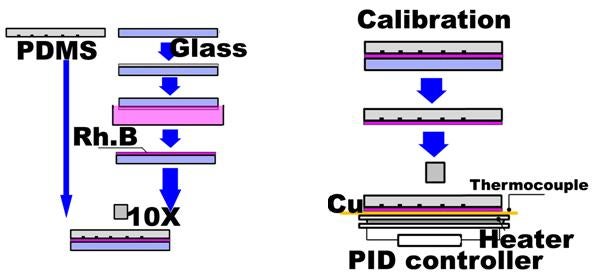
Chip fabrication procedure and temperature measurement calibration.
In this method, a fluorescent polymer film (PDMS saturated with Rhodamine B) is introduced as one layer of a microfluidic chip (pink layer above) directly bonded with the substrate with a microchannel in it. The principle of measurement is to retrieve the chip temperature profile by monitoring the temperature-dependent photobleaching speed of the thin fluorescent polymer film.
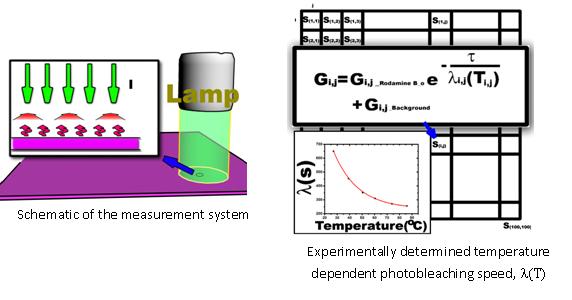
Comparison between numerically (left) and experimentally (center) predicted chip temperature profile. The higher temperatures outside of the channel region measured in the experiments are due to manufacture defects (right). The gap between chip substrates near the channel walls is filled with the working liquid which has Joule heating and is the heating source in this experiment. This is the reason causing higher temperature in measured chip temperature profile, but not shown in numerical results which assumes perfect channel features.
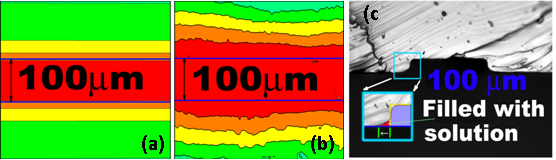
Method II:
This method utilizes a similar chip structure as that in Method I. The principle of the measurement is to measure the temperature-dependent fluorescent intensity of the thin film which will lead to the chip temperature profile. The calibration curve of the thin film is as shown in the figure below.
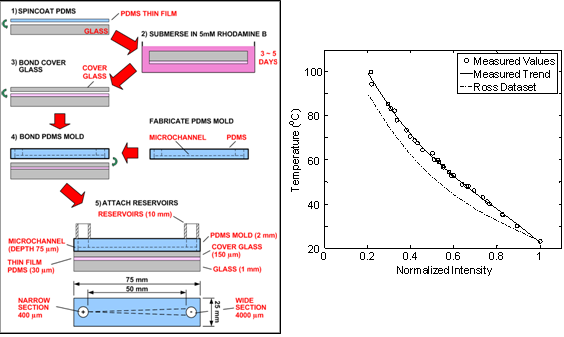
Comparison of the experimental temperature profile along the tapered channel for 400mW to the results from the numerical model. Also included are the numerical temperature profiles at the top, middle and bottom of the PDMS thin-film.
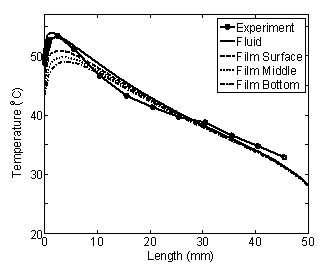
Method III:
Method I and II have advantages in measuring temperature profiles for the entire chip, however, these two methods can not measure the channel temperature directly. Ross' method [1] allows the channel temperature to be measured directly if the above-mentioned absorption and adsorption problems can be resolved. This method aims to improve the measurement accuracy of Ross' method by reducing the fluorescent noise contributed from the absorbed and adsorbed dye particles in the surrounding chip material, which can be done using photobleaching.
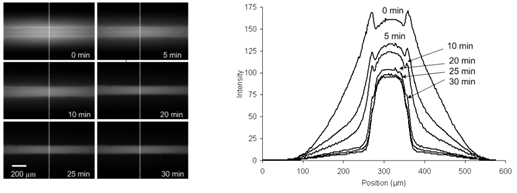
Sequence of fluorescent images acquired during the course of photobleaching a PDMS microchannel with a 100µM solution of Rhodamine B pumped at 20µL/min (left) and corresponding light intensity profiles showing the decrease in signal from absorbed Rhodamine B (right).
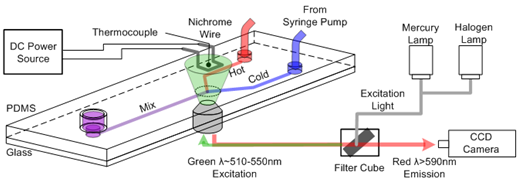
System used for photobleaching.
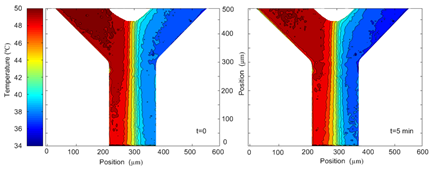
Temperature contour plots of the intersection obtained from two images taken 5 minutes.
Bibliography
T. Glawdel, C. L. Ren, “Photobleaching absorbed Rhodamine B to improve temperature measurements in PDMS microchannels (PDF)”, Lab Chip, 9 (2009) 171-174.
L. Gui, C. L. Ren, “Temperature Measurement in Microfluidic Chips Using Photobleaching of a Fluorescent Thin Film (PDF)”, Applied Physics Letters, 92 (2008), 024102.
R. Samy, T. Glawdel, C. L. Ren, “Method for Microfluidic Whole-Chip Temperature Measurement Using Thin-Film Poly(dimethylsiloxane)/Rhodamine B (PDF)”, Anal. Chem., 80 (2008), 369–375.