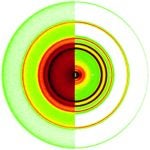
Velocity map imaging, or VMI, has become the technique of choice for many chemical dynamics research laboratories. VMI is analogous to time-of-flight mass spectrometry, the main difference being that through judicious choice of electric fields in the ion source region one is able to direct ions with the same mass and initial velocity to the same position on an ion detector.
By exciting a molecule or cluster with laser light we are able to induce dissociation quantum-state selectively. Any energy delivered by the photon that is in excess of the dissociation threshold is then partitioned between the kinetic and internal energies of the nascent particles. The newly formed product species expand on a Newton sphere defined by conservation of momentum in the photodissociation process. When crushed onto the 2D detector plane, these spheres form circular images like the one shown on the left. By measuring the image radius, and the particle mass and time-of-flight, we can calculate particle kinetic energies. Since we know what photon energy our laser is delivering, we can use straight-forward energy accounting to determine the bond strengths of the dissociation molecules. If more than one product channel is accessed, we can also measure the relative probabilities or branching ratios, for each channel. The picture on the left shows the Au velocity map image acquired following Au2 photodissociation at 157 nm.
VMI of Rare Gas Dimers
In my lab, we have built a VMI instrument that is capable of producing and studying van der Waals-bound dimers of rare gases. The image below shows a schematic of our VMI apparatus. We can operate in time-of-flight mode to conduct resonance enhanced multiphoton ionization (REMPI) experiments or in VMI mode to record photofragment images, which we can analyse to garner insight into excited state dynamics.
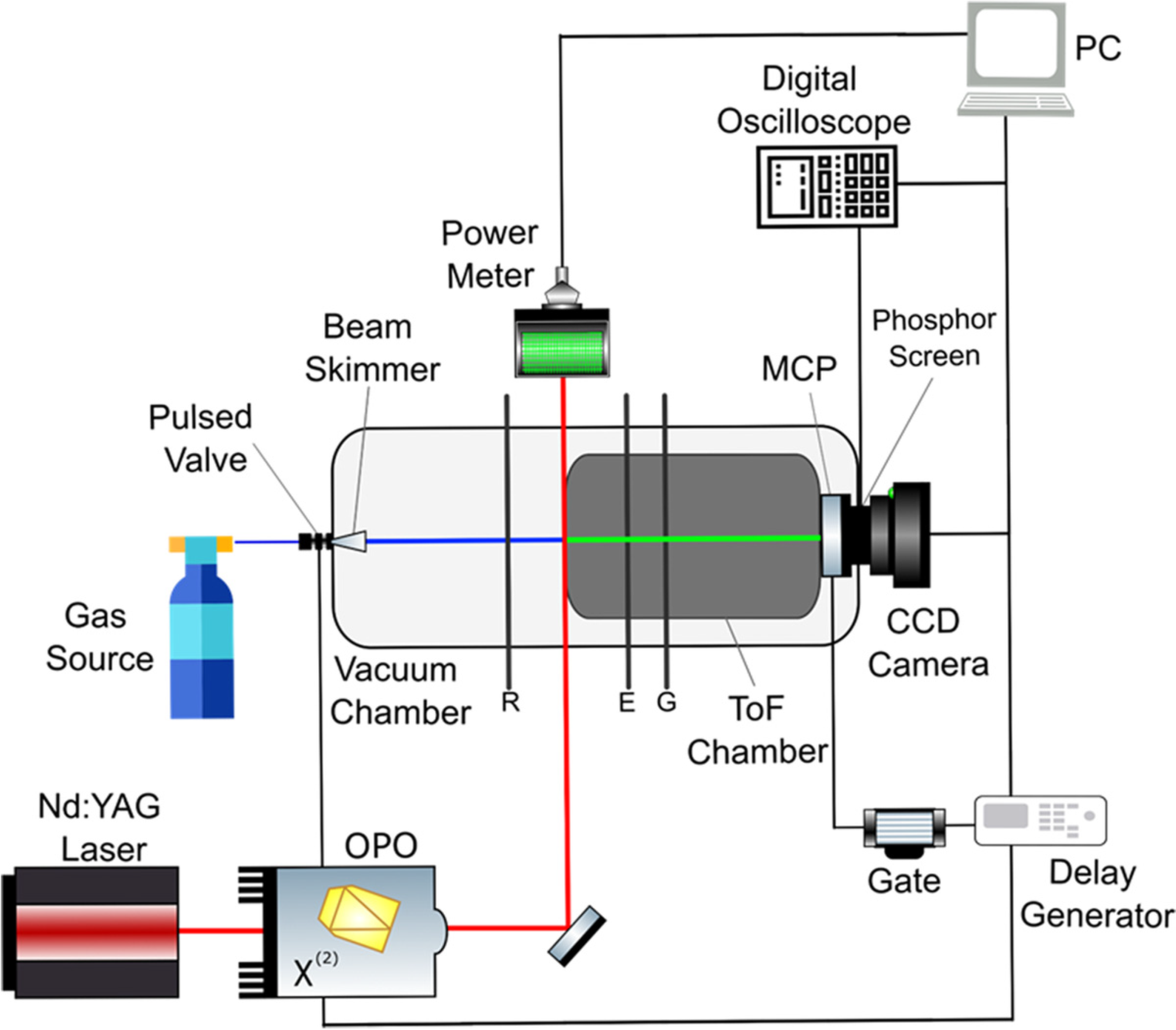
When conducting REMPI spectroscopy of Kr2, we can observed vibronic (i.e., vibrational-electronic) transitions in the Kr2+ and Kr+ mass channels. A section of the Kr2 (2+1) REMPI spectrum is shown below. The peaks are associated with transitions from the vibronic ground state to excited Rydberg states at the two-photon level. Observation of Kr+ indicates that photofragmentation of the excited state competes with absorption of a third photon to induce photo-ionization. Details of our analysis can be found at this link.
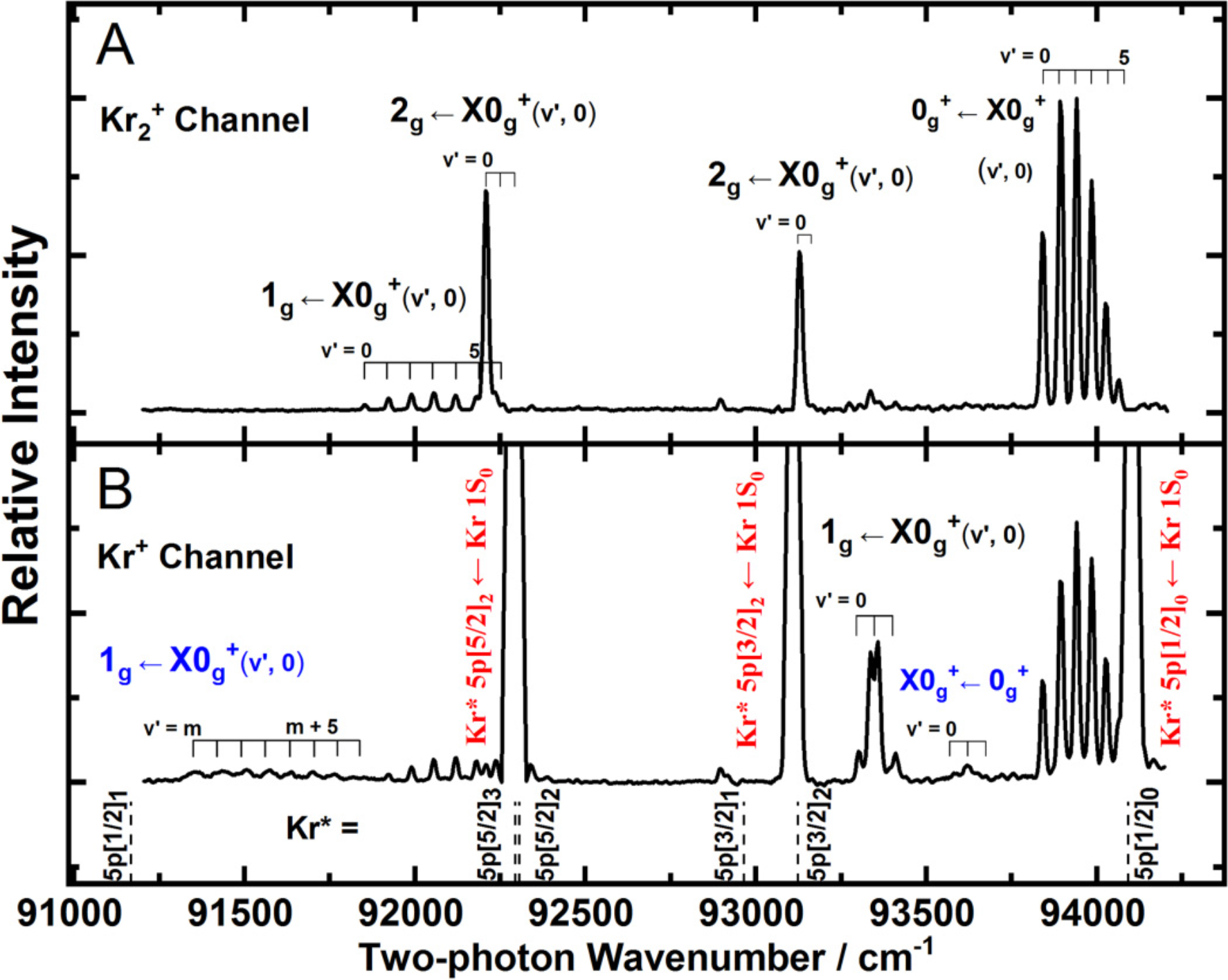
Images of the Kr+ photo-product yield information on dissociation energies, product branching ratios, and angular anisotropies, which inform us as to which quantum states are accessed during the photo-excitation and dissociation process. The Kr+ image shown below was recorded following excitation to the Kr2 5p [5/2]2 1g Rydberg state. Panels A and B show the ion intensity distribution and panel C plots the intensity as a function of angle relative to the electric field of the plane-polarized dissociation laser. The anisotropy parameters (beta values) correlate with the symmetries of the quantum states involved in the photo-excitation and dissociation process, and with the excited state lifetime.
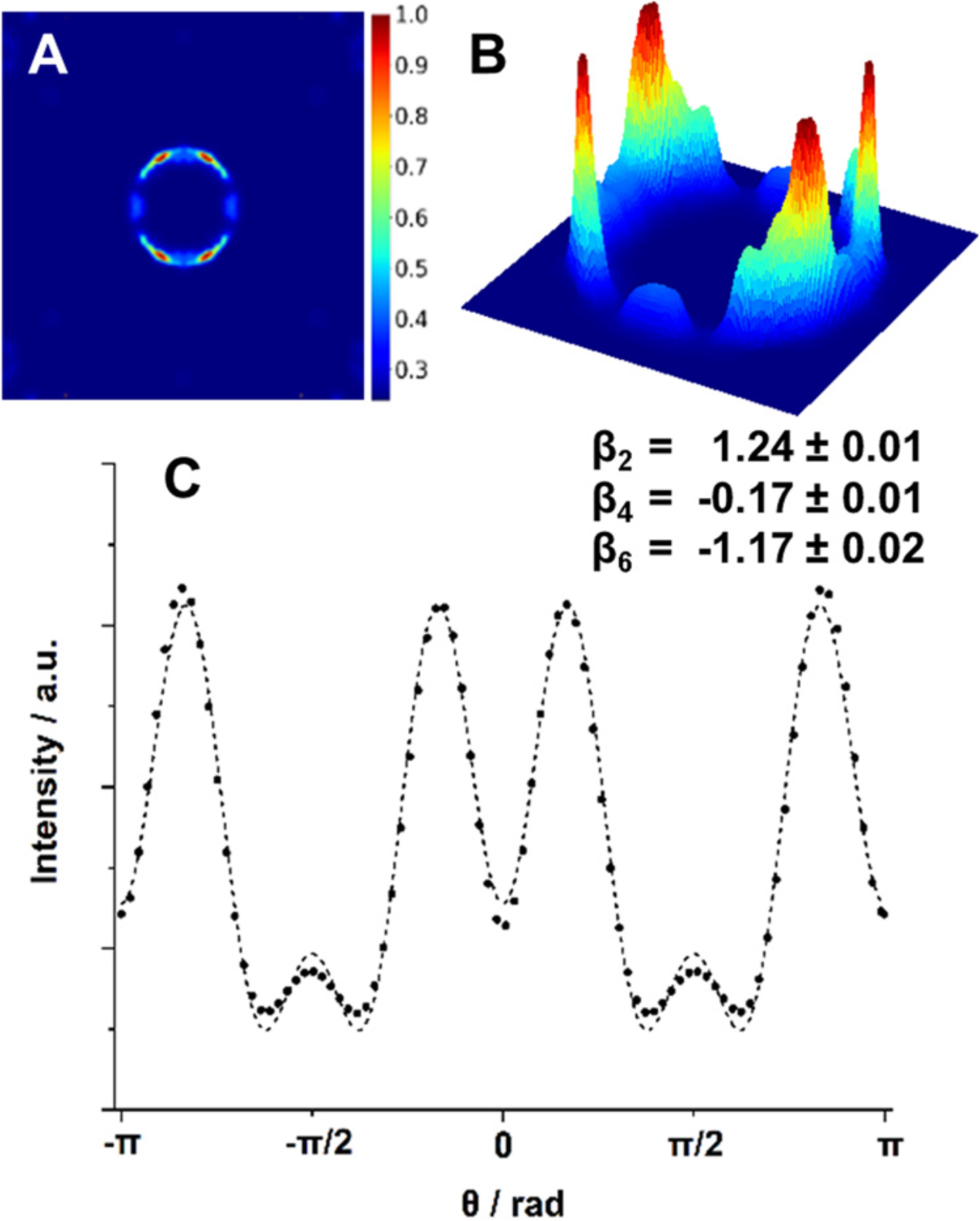
By plotting the total kinetic energy release (TKER) of the observed photofragments as a function of excitation energy, we can identify linear trends that tell us to which dissociation thresholds products correlate (see below). A global fit of all products observed from all vibronic transitions gives us an excellent measurement of bond dissociation energies for the ground and excited states.
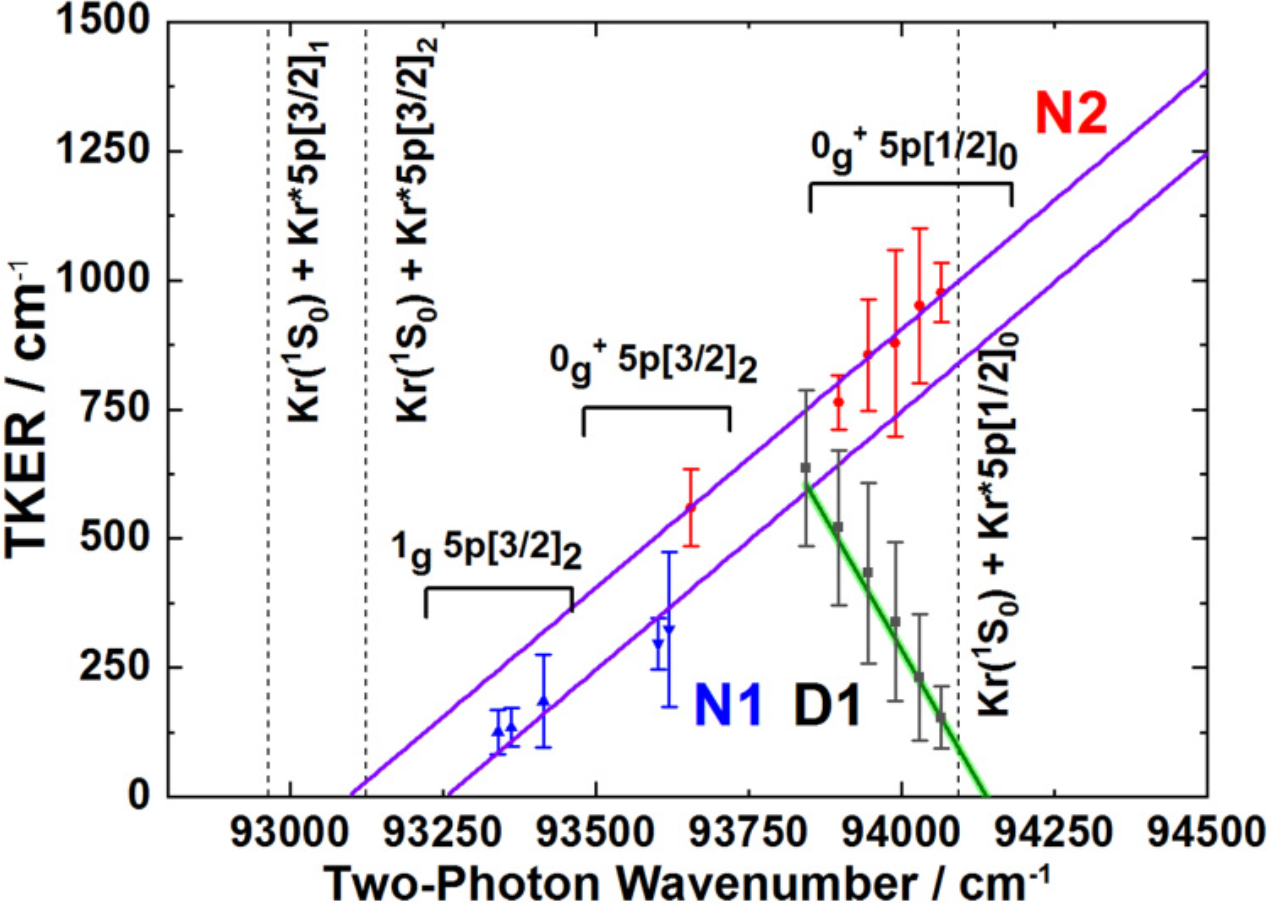