IQC researchers propose two possible methods for building an optical cavity inside a hollow-core on-chip waveguide, to develop a platform for quantum information processing through photon-photon interactions.
Particles of light, called photons, can carry information over long distances and make excellent qubits for quantum communication. However, photons generally do not “talk” to each other – in other words, photons do not interact with other photons on their own. This makes quantum logic gates, one of the building blocks of a quantum computer, difficult to implement for single photon operation.
At the same time, atoms and photons do “talk”. A photon can change the state of an atom, while an atom can absorb or change the phase of a photon depending on the atom’s state. If two photons interact with the same atom, this can result in an effective photon-photon interaction. The technological challenge for scalable quantum information processing or other applications is then creating an environment where controlled light-matter interaction can occur on a chip, which requires simultaneous tight confinement of both photons and atoms.
One approach has been loading and trapping laser-cooled atoms, which are better at interacting with single photons than their room-temperature counterparts, inside hollow-core waveguides for tight transverse confinement. Integrating a pair of mirrors into the waveguide to form a cavity adds longitudinal confinement for light to this platform.
Forming mirrors inside conventional, solid-core optical waveguides, such as optical fibres, is commonly done by periodically modulating the refractive index of the waveguide’s core along its length. When the period of modulation is chosen correctly, this can create highly reflective mirrors. These mirrors operate on a principle similar to anti-reflection coating on glasses or camera lenses, except they act as anti-anti-reflection coatings and can achieve reflectivity as high as 99.999%. However, since the hollow core of the waveguide does not offer any material for modulation, adding mirrors is tricky without clogging up the waveguide.
In their exploratory work recently highlighted by OSA Publishing’s Spotlight on Optics, Master’s student Golam Bappi, with PhD students Jeremy Flannery, Rubayet Al Maruf and faculty member Michal Bajcsy present two methods for fabricating mirrors in a hollow-core on-chip waveguide to facilitate photon-photon interactions.
“The waveguides we study have hollow cores for introducing cold atoms that have been cooled to micro-Kelvin temperature,” explained Bajcsy, who is also an assistant professor in the Department of Electrical and Computer Engineering at the University of Waterloo. “Eventually the atoms warm up and need to be replaced with cooled atoms again, so maintaining access to the hollow core of the waveguide is important.”
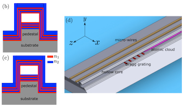
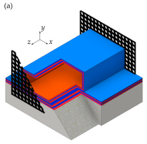
Bajcsy’s Nano-Photonics and Quantum Optics Lab has already started the fabrication of the proposed structures. The next steps will be to evaluate their performance in an experimental set-up where cold atoms are prepared and loaded in to the waveguide, and photons are sent in for interaction.
In addition to the development of quantum logic gates operated with single photons, other potential applications of these structures include optical transistors in which the flow of light is controlled by a single photon and precision lasers, called superradiant lasers, useful in precision metrology.
The paper Prospects and limitations of bottom-up fabricated hollow-core waveguides appeared in Optical Materials Express in January.