The concepts of a quantum vacuum and quantum vacuum fluctuations are still not accepted by everyone. However, a group of researchers including Christopher Wilson of the Institute for Quantum Computing have found further evidence that the two concepts are a reality. Through experiments conducted at Chalmers University of Technology, the researchers were able to probe the quantum vacuum fluctuations and not only measure their strength, but also map out their shape them.
Using a mirror, the researchers were able to engineer the vacuum to reduce the fluctuations that in turn, control the vacuum modes, or give the fluctuations a well-defined shape. In many situations, the vacuum fluctuations limit the coherence, or lifetime, of a quantum bit (qubit) – in this case a superconducting artificial atom. If there were no vacuum fluctuations to cause the decay of the atom, it would stay in the excited state. Studying the lifetime of the atom therefore allowed Wilson and colleagues to probe the vacuum fluctuations.
“This is important from a quantum computing aspect,” said Wilson, an associate professor in both the electrical and computer engineering and the physics and astronomy departments at the University of Waterloo. “By figuring out different ways to shape and control the vacuum fluctuations, it’s a step towards the goal of developing quantum technologies.”
Similar experiments have been done before with “real” atoms and mirrors, however those results weren’t able to map out the structure of the fluctuations very well. When using atoms, it’s difficult to aim all the radiation from the atom at the mirror and the motion of the atom is also problematic. In the experiments at Chalmers, a more controlled approach was achieved by using engineered artificial atoms.
Microwaves, which are electromagnetic radiation, went in and out via wires attached to the transmission line on a chip of aluminum, a superconducting metal (see the figure below). The line was short-circuited at the other creating the equivalent of a mirror. By actively modifying the response of the qubit to different microwave frequencies, and measuring its lifetime, the researchers were able to probe the strength of the vacuum fluctuations.
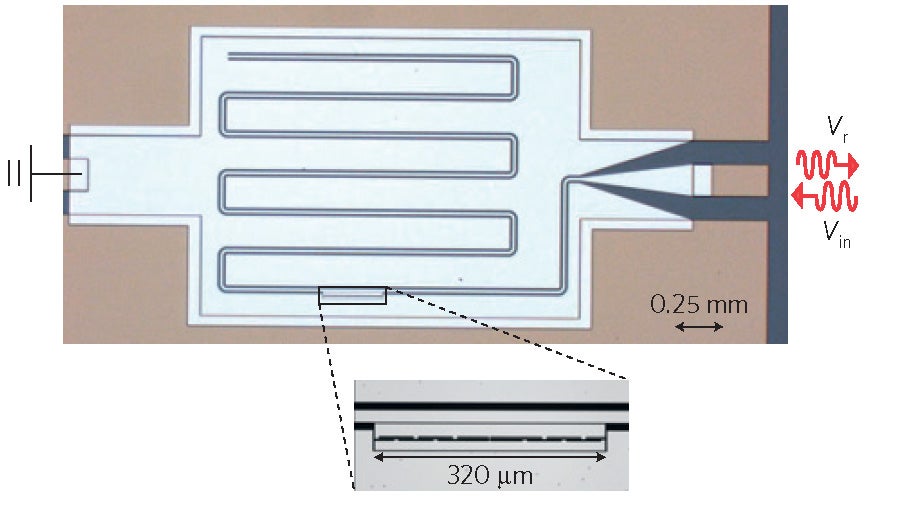
Unlike previous experiments using real atoms, a superconducting qubit is fixed and doesn’t move, which allows for a clearer mapping of the spatial structure of the vacuum fluctuations.
Though constricted by the waveguide, the superconducting qubit was also hanging out in open space. In previous research, real atoms were placed in a cavity – it’s like putting it in a little box so it’s completely isolated. By placing the atom in a cavity, it retains its coherence longer because it limits the interaction with the environment. In this experiment, even though the superconducting qubit was in open space, the interaction with the mirror kept the qubit hidden from the vacuum fluctuations, allowing it to stay coherent longer.
By not placing the atom in a box, the researchers could explore the spatial construct of the vacuum, which will be helpful for quantum technologies. Take quantum communications for example. If you have an atom trapped in a box and want it to interact with light, the photons also have to be in the box. For the purpose of communications, you want the photons to move around to send between people, not locked in a box, so if the atom is in open space it can then interact with the photons that are moving around.
The paper, Probing the quantum vacuum with an artificial atom in front of a mirror, was published in Nature Physics, September 28, 2015. It was part of a series of experiments looking at atoms in open transmission lines that began when Wilson was an assistant professor at Chalmers.