In a paper published in Physical Review Letters, PhD student Sascha Agne and colleagues experimentally realized a three-photon Greenberger-Horne-Zeilinger (GHZ) interferometer and observed genuine three-photon interference for the first time, bringing scientists one step closer to exciting applications in quantum communication.
The work builds on previous research by faculty members in the Department of Physics and Astronomy Thomas Jennewein and Canada Research Chair in Optical Quantum Technology Kevin Resch. They demonstrated the first direct generation of photon triplets in 2010, and time-energy entanglement in three photons in 2012. These time-energy entangled photon triplets are physically interesting, but difficult to access experimentally.
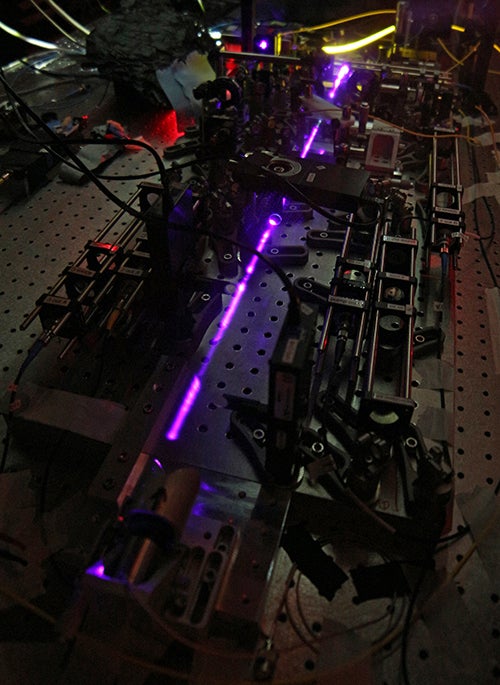
To explain, Agne uses the analogy of three people in a room. If all three people are doing the same thing, we can say their behaviour is correlated, or in quantum mechanical terms, entangled. Normally, this means any two of these people also have correlated behaviour. In the bizarre world of quantum mechanics however, entanglement between three (or more) particles can create correlations between all the particles, not any individual or pair separately. The researchers translated this abstract reality into a tangible interference pattern that has many possible applications.
One such possibility is a protocol called secret sharing. Imagine a trio of people who each have to use their thumbprint to unlock a safe. One or two of those people cannot secretly open the safe, as the third person is necessary. This latest research opens the door to implementations of this idea in quantum cryptography.
Another important outcome of the experiment is the high interference visibility achieved. Visibility is a measure of the quality of control the researchers have over the phase of the interferometer. Using classical light fields, the upper bound of the visibility of three-photon coincidences is 50 percent, but in quantum mechanics, 100% is possible. The researchers achieved well over 90 percent, leaving no doubt they measured a quantum mechanical effect.
These extraordinary results were made possible by a culmination of technological improvements, including the photon triplet source from Jennewein and Resch, new superconducting nanowire detectors, and high-resolution time tagging devices by Jennewein.
The experiment is a result of a collaboration led by Jennewein, between IQC and Department of Physics and Astronomy researchers Sascha Agne, Jeongwan Jin, Resch and Jeff Salvail, former IQC members Gregor Weihs, Evan Meyer-Scott and Deny R. Hamel, and University of Innsbruck researcher Thomas Kauten.
Agne hopes to expand their experimental setup in the future. “In our experiment, energy and time are continuous, not chunked, because the photon triplets were generated using a continuous wave laser. If we were to generate these triplets using what we call time bins, essentially pulsed lasers, we would end up with a discrete version of time-energy entanglement, which is more immediately useful in quantum communication.” Quantum communication over optical fibres, for example, requires time bin encoding. Discrete time-energy entanglement has been achieved in two photons, but not three, which could have distinct advantages in quantum communication networks.
A research group led by Ian Walmsley, Pro-Vice-Chancellor for Research and Hooke Professor of Experimental Physics at the University of Oxford, observed a similar phenomenon in an independent experiment at the same time as Ange’s group. After making contact, both groups decided to jointly submit their work to Physical Review Letters, and the journal published a story on this accidental convergence.