Robert Hill
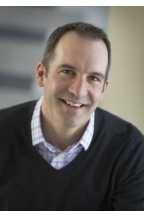
Associate Professor, Teaching Fellow
Email: robhill@uwaterloo.ca
Location: PHY 248
Phone: 519-888-4567 x46844
Biography
Dr. Hill's research is focused on the experimental study of materials whose exotic properties are dominated by the collective quantum mechanical nature of their electrons and defy explanation using current theoretical paradigms.
The difficult challenge of predicting emergent collective electronic properties means that such search and discovery is lead by experiment.
Dr. Hill's research provides the precise low temperature measurements of fundamental properties which form the benchmarks against which theoretical understanding is tested.
The difficult challenge of predicting emergent collective electronic properties means that such search and discovery is lead by experiment.
Dr. Hill's research provides the precise low temperature measurements of fundamental properties which form the benchmarks against which theoretical understanding is tested.
Research Interests
- Unconventional superconductivity
- Quantum phase transitions
- Transport in low dimensional materials
- Quantum Science
Scholarly Research
Superconductivity, where electricity flows without any resistance, is a dramatic example of the collective quantum mechanical behaviour of electrons in materials.
It has the capacity to revolutionize current and future technologies, although, at this point in time, widespread commercial use is unfeasible because it remains a property that is only exhibited at low temperatures. This provides such a significant impediment to its use that society has yet to harness its real potential.
Dr. Hill's goal is to develop a deeper understanding of this phenomena. Examples of specific knowledge that need to be accrued include:
How can the superconducting transition temperature be increased?
What possible mechanisms can be used to couple electrons to form Cooper pairs?
Are there alternative routes to superconducting states beyond forming Cooper pairs of electrons?
Such challenging questions are difficult to answer directly. However, they may be addressed by assembling information assimilated from interpreting measurements of the fundamental properties of novel superconducting materials. Ultimately, this knowledge can then be used to help to engineer the next generation of superconducting materials with, for example, elevated transition temperatures.
Nowhere are the conventional paradigms of condensed matter theory challenged more than in systems whose properties are governed by quantum critical phenomena.
For example, the central assumption of Landau’s Fermi-liquid theory is that electrons in metals should behave as a weakly interacting collection of electronic quasiparticles. One situation in which the properties of materials cannot be explained using this approximation is in the proximity of a quantum critical point.
A quantum critical point occurs when a non-thermal control parameter is used to suppress a phase transition to the absolute zero of temperature. At this point the phase transition is driven by quantum fluctuations due to the Heisenberg uncertainty principle as opposed to thermal fluctuations. In practice, the non-thermal control parameter is pressure (either externally or chemically applied) or a magnetic field. A dramatic feature of this apparent T= 0 K phenomenon is that the presence of the quantum critical point leaves its fingerprints on the behaviour of the system at temperatures many decades higher. As a consequence, quantum criticality has been suggested to be the underlying physics driving the properties of such diverse systems as the high temperature cuprate superconductors or even the universe itself, with the whole of existence sitting on the verge of a second order phase transition.
In the absence of a microscopic theory to describe behaviour at a quantum critical point, a picture of the phenomenology is starting to emerge, although many challenges remain. In particular it is crucial to be able to distinguish the effects of intrinsic physics from disorder. Many of the materials that have been studied, especially in the chemical pressure studies, are non-stoichiometric, and as such are prone to such ambiguities. Our research program ensures excellence by using magnetic field as the tuning parameter and concentrating on well-characterized samples. One can also study the effects of a quantum phase transition in the absence of itinerant electrons using the transverse field Ising model. The transverse magnetic field suppresses the magnetic ordering temperature to zero kelvin, whereby the phase transition between the order and disordered phases is controlled by quantum fluctuations.
In the case of novel magnetic systems, the research is primarily driven by looking for new and emergent physics resulting from geometrical frustration of the magnetic moments. The near equivalence in energy of many magnetic states in the presence of frustration makes the theoretical prediction of magnetic behaviour an extremely difficult task and hence experiment plays a pivotal role in this area of research. One type of phase of particular current interest is that of a spin liquid, an insulating magnetic phase that has no long-range magnetic order. Our objective is to experimentally identify spin liquid states, with the distinct possibility of uncovering qualitatively new states of matter in these complex systems.
Another interesting state is that adopted by the "spin-ice" materials (Ho2Ti2O7 and Dy2Ti2O7). The spin configuration imposed by frustration leads to a residual entropy analogous to that seen in (water) ice. The collective excitations of this magnetic system are also thought to have the properties of magnetic monopoles. Our research aims to take advantage of this unique opportunity by studying the transport properties of these infamous elementary excitations.
Education
- 1996, Doctorate Physics, University of Bristol, Bristol, United Kingdom
- 1991, Bachelor of Science (BSc) Physics, University of Bristol, Bristol, United Kingdom
Service
- Associate Dean of Graduate Studies, Faculty of Science, University of Waterloo
Teaching*
- PHYS 111 - Physics 1
- Taught in 2019, 2020, 2021, 2022, 2023
- PHYS 233 - Introduction to Quantum Mechanics
- Taught in 2019, 2020, 2021, 2022, 2023, 2024
* Only courses taught in the past 5 years are displayed.
Selected/Recent Publications
- WH Toews, RW Hill. "A robust and well shielded thermal conductivity device for low temperature measurements". Review of Scientific Instruments 85 (4), 043905 (2014)
- WH Toews, SS Zhang, KA Ross, HA Dabkowska, BD Gaulin, RW Hill. "Thermal Conductivity of Ho2Ti2O7 along the [111] Direction". Physical review letters 110 (21), 217209 (2013)
- Johnpierre Paglione, MA Tanatar, DG Hawthorn, Etienne Boaknin, RW Hill, F Ronning, M Sutherland, Louis Taillefer, C Petrovic, PC Canfield. "Field-Induced Quantum Critical Point in CeCoIn5. Physical review letters 91 (24), 246405 (2003)
- R.W. Hill. "Superconducting Levitation". Physics in Canada 67, 136 (2011)