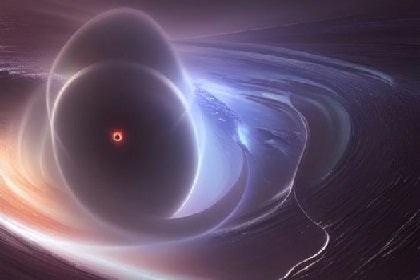
Black holes are formed when extremely massive objects like stars collapse to a singularity – a point of infinite density. The gravitational field of a black hole is so great that nothing can escape its clutches, not even light. This creates a spherical region of space around the singularity entirely cut off from the rest of the universe and bounded by what is known as an event horizon.
An active area of research into the physics of black holes seeks to develop a consistent theory of quantum gravity. This is an important goal of theoretical physics that would reconcile quantum mechanics and Einstein’s general theory of relativity. In particular, by considering black holes in quantum superposition, physicists hope to gain insights into the quantum nature of space–time.
Unruh–deWitt detector
In the latest work, reported in Physical Review Letters, Joshua Foo and Magdalena Zych of the University of Queensland together with Cemile Arabaci and Robert Mann at the University of Waterloo outline what they describe as a new operational framework for studying space–time superpositions. Rather than using a “top-down” approach to quantize general relativity they instead consider the effects of a black hole’s quantum state on the behaviour of a specific physical device called an Unruh–deWitt detector.
This is a hypothetical device that comprises a two-state system, such as a particle in a box, coupled to a quantum field. When in its low-energy state and exposed to electromagnetic radiation of just the right frequency, the system jumps to its higher state and registers a “click”.
This kind of detector can in theory be used to measure Unruh radiation, a heat bath of particles that is predicted to appear from the quantum vacuum to an observer that is accelerating through space. In the scenario laid out in the new research, it would instead capture Hawking radiation. This is radiation that is predicted to be created when virtual particle–antiparticle pairs within the quantum vacuum are ripped apart at a black hole’s event horizon – the antiparticle then disappearing into the void and the particle emitted into the surrounding space.
In their thought experiment, the quartet envisage an Unruh–deWitt detector located at a specific point outside a black hole’s event horizon, with the detector’s fixed position enabled by an acceleration away from the black hole that yields the Hawking radiation. The researchers consider the effect of a superposition of the black hole’s mass on the output of that detector.
Superposition of distances
As they explain, the two masses yield different solutions to the field equations of general relativity and thereby distinct space–times. The resulting superposition of space–times in turn leaves the detector in a superposition of distances from the event horizon, creating what is in effect an interferometer whose arms are each associated with one of the black hole masses. The probability that the detector clicks depends on which masses are present in the superposition.
Doing the calculations for a relatively simple black hole described in two spatial dimensions by the Banados–Teitelboim–Zanelli formulation, the physicists obtained a striking result. They plotted the probability of detecting a particle emitted by the black hole as a function of the square root of the superposition mass ratios and found sharp peaks when those values were equal to 1/n, with n being an integer.
“This result provides independent support for Bekenstein’s conjecture,” the researchers write in Physical Review Letters, “demonstrating how the detector’s excitation probability can reveal a genuinely quantum-gravitational property of a quantum black hole”.
The four physicists stress that the result emerged from their calculations without assuming that the black-hole mass had to fall within the discrete bands predicted by Bekenstein’s conjecture. They add that their technique could be extended to more complex descriptions of black holes in three spatial dimensions, which they say, would provide additional insights regarding the effects of quantum gravity in our universe.