Introduction
Crash testing is a group of tests that allow for the evaluation of the deformation mode and energy absorption characteristics of lightweight structural components. There are several types of structural testing: axial crush, side impact and oblique testing. Furthermore, components can be crushed at high speeds (called dynamic crashing) and at low speeds (called quasi-static crashing). Types of components that have been crushed include: hot stamped B-pillars, tailored hot stamped rails, brake-formed DP980 rails, tailor-welded axial crush rails, warm formed 6000 and 7000 series aluminum rails and hot formed 7000 series aluminum rails.
In addition to physical testing, the dynamic and quasi-static experiments are modelled using finite elements. Finite element modelling.
Structural Crashworthiness Crash Sled Facility
The University of Waterloo Structural Crashworthiness Crash Sled Facility consists of a Seattle Safety impact sled, with a maximum available impact energy of 169,500 J. Maximum allowable payload is 1,400 kg. The sled size is 2,500 mm x 1,200 mm and can accommodate a payload of up to 1,400 kg.
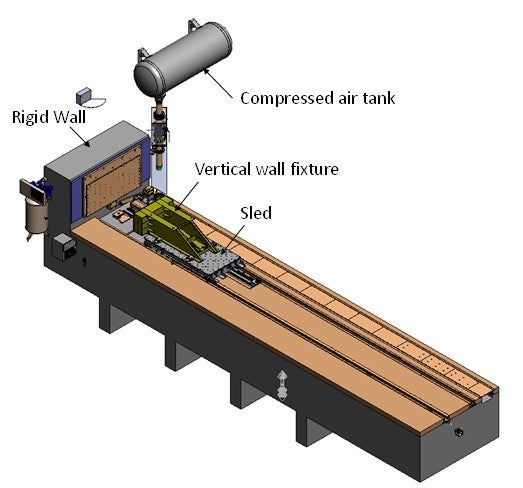
Data Acquisition
- The sled data acquisition system is capable of recording loads, accelerations, high speed video and high speed infra-red video.
- Six Kistler Quartz Force Link (model #9371B) load cells rated for ± 120 kN are available for force measurements. The load cells can be mounted on the wall or on the sled.
- Sled acceleration is measured using two MSI accelerometers (model # 64B-0100-004) mounted on the front of the sled. The load cell and accelerometer data are recorded using on board and wall mounted DTS Slice data acquisition systems.
- High speed video of the tests can be recorded using up to three Photron high speed cameras (one SA-4 and two SA-5s), which are capable at recording at frame rates in excess of 100,000 frames per second (fps). Typically, tests are recorded at 5,000 fps which provides high definition quality video of the tests.
- A Telops FAST-IR 2K thermal camera is available for high speed infra-red video.
Experimental Setup
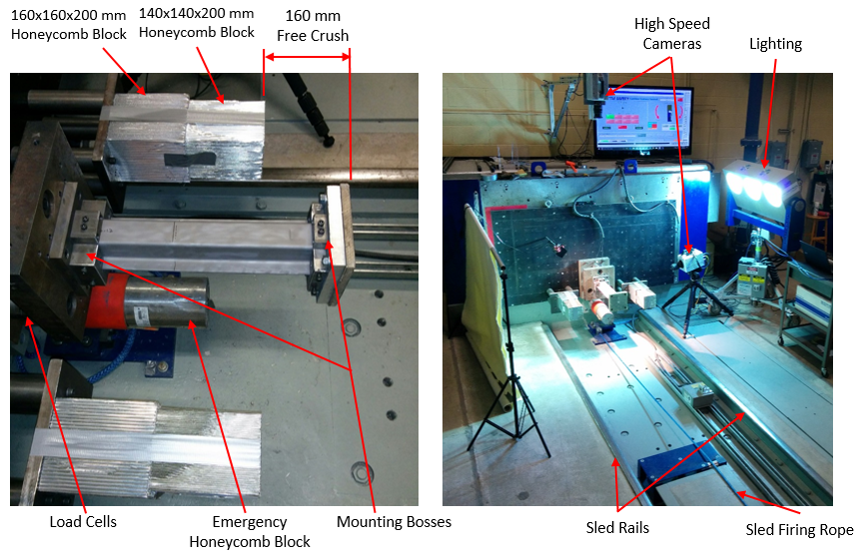
Dynamic crash setup for axial crush
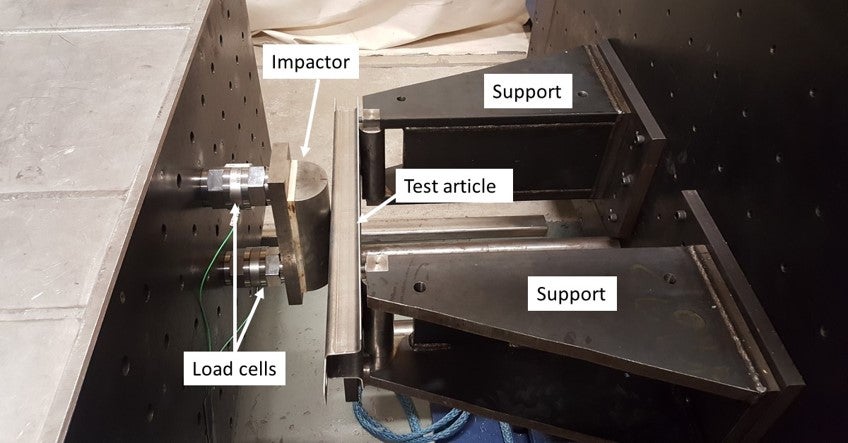
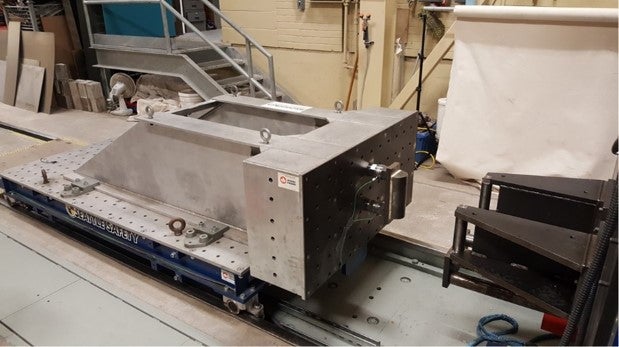
Dynamic crash setup for three-point bends
Testing of Production Components
The impact can be used to test experimental samples as well as production components. The image below shows an experimental set up for a production front rail section. Three Photron high speed cameras were used to record the deformation, together with a Telops high speed IR camera.
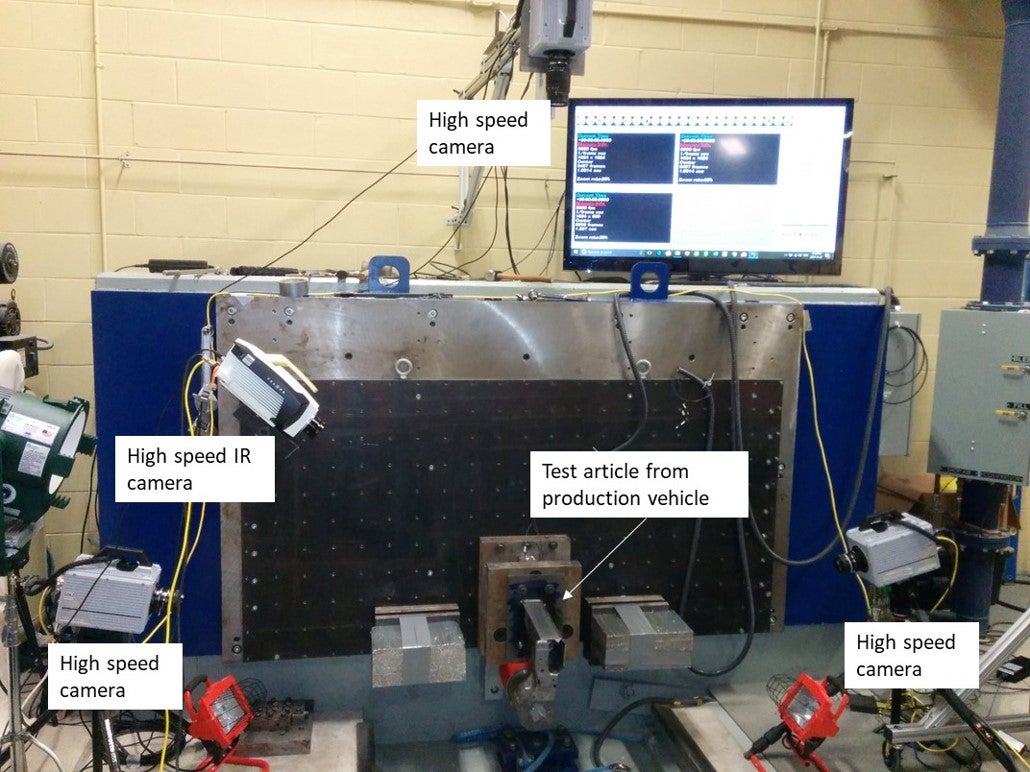
Quasi-static Validation
Quasi-static experiments are performed on a hydraulic actuator shown below. Dynamic crush experiments are performed on a Seattle Safety D780-3.7 crash sled at the University of Waterloo.
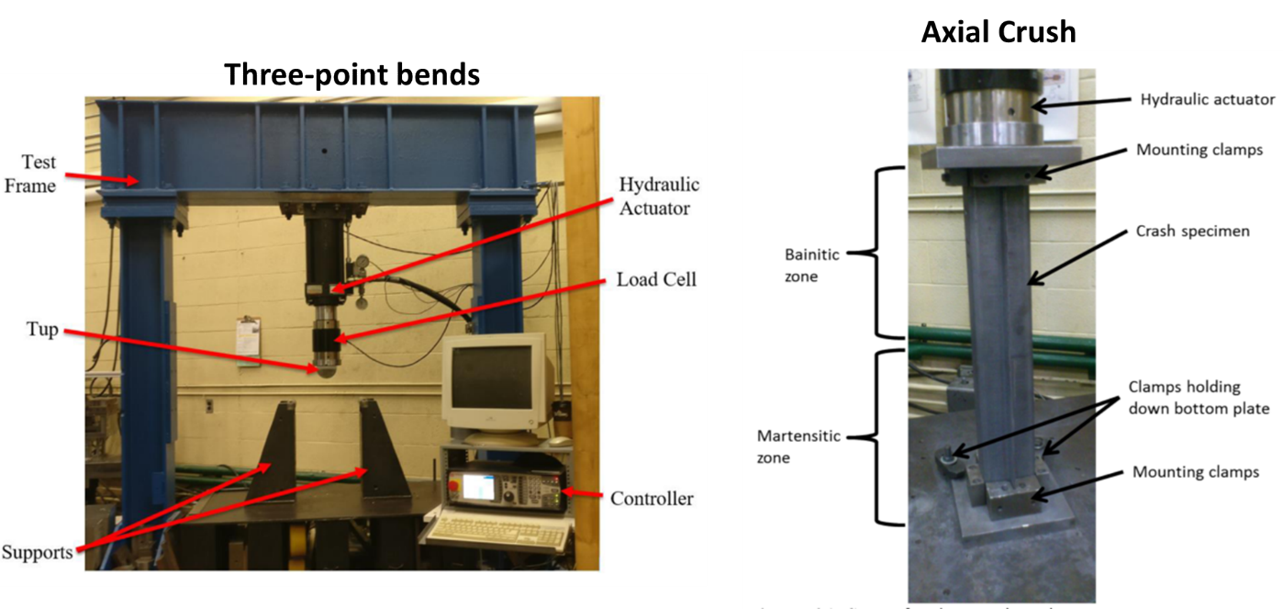
Quasi-static crash setup for three-point bends and axial crush
Finite Element Modelling
All crash experiments are modelled in LS-DYNA, which is a commercially available finite element code.
Crash models make use of the high speed constitutive data and fracture data and apply them onto structural assemblies.
B-Pillar
A lab-scaled B-pillar was hot stamped and subject to dynamic three-point bend tests.
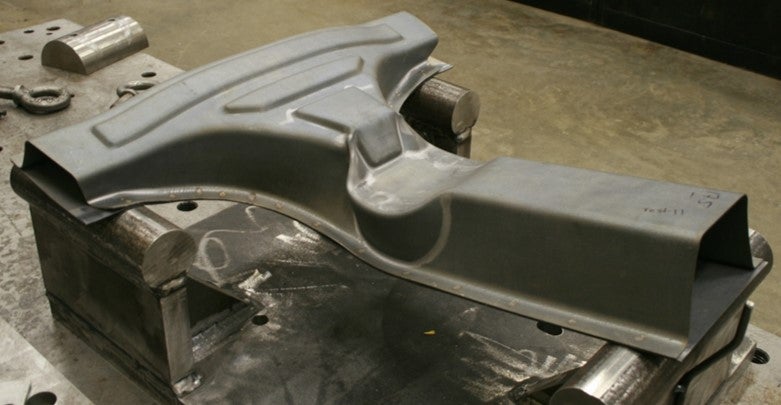
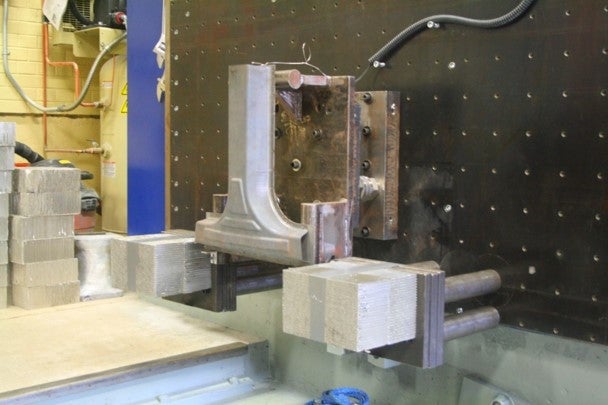
Left: Testing a B-Pillar component on the crash sled. Right: B-Pillar after crash testing
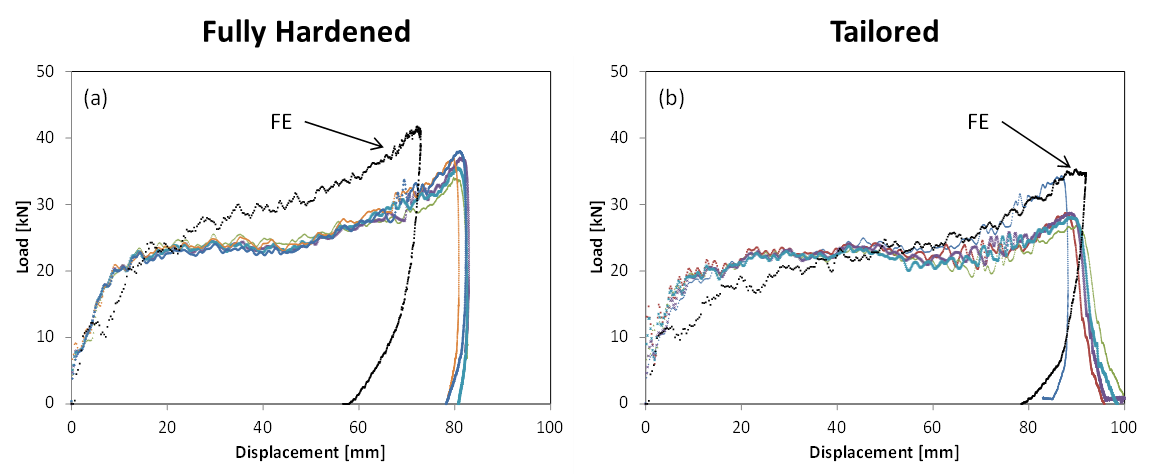
Fully hardened and Tailored crash response (Force vs. Displacement). FE Models and Experiments
B-pillar crash videos of the sled and finite element model
Tailored Hot Stamped Rails
Tailored hot stamped rails were crushed in two ways: side impact (where only the flanges were tailored) and axial crush (where the entire body was tailored). For side impact, tailoring was shown to improve the ductility of the material at no significant cost to energy absorption of load displacement. For axial crush, the part exhibiting a graded soft zone experienced a folding pattern during crush, with little to no buckling, and a favourable energy absorption response.
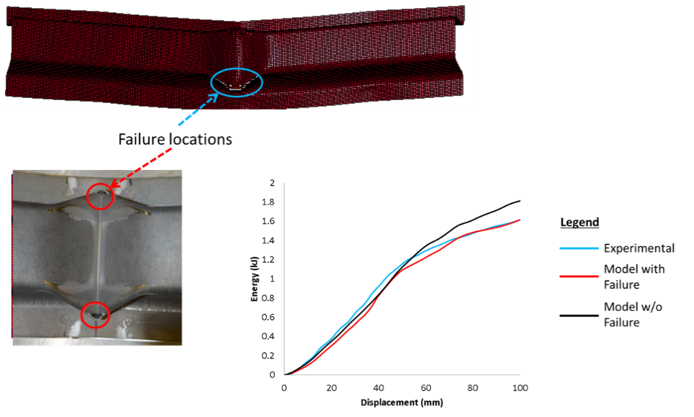
Side impact results for fully quenched hot stamped rails

Side impact results for tailored hot stamped rails
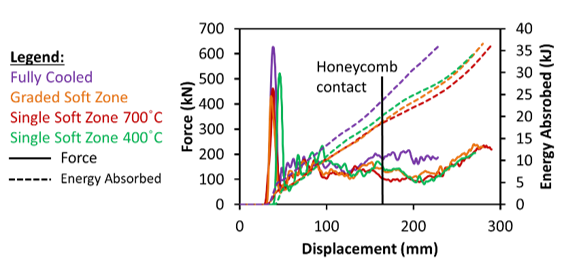
Axial crush energy absorption
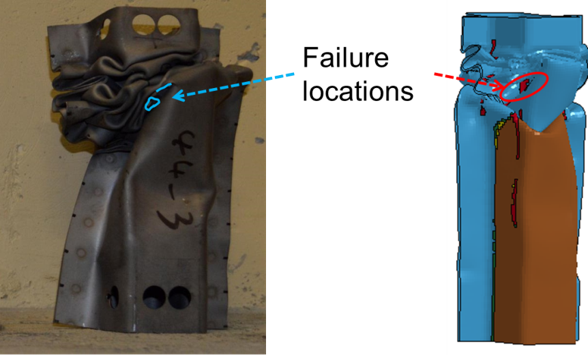
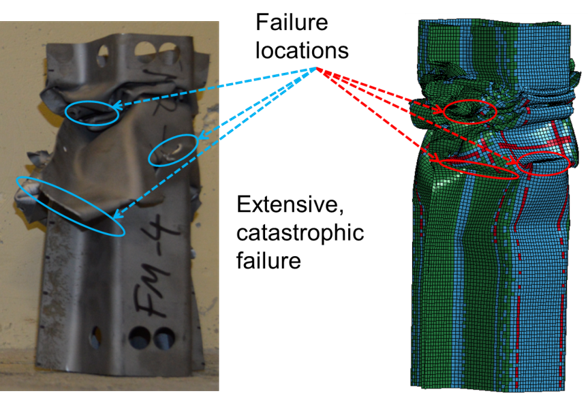
Axial crush experiments and model predictions for (a) a fully quenched rail and (b) a tailored rail
Tailor Welded Blanks
Similar to the tailored hot stamped rails, tailor welded top hat-shaped channels were formed and subsequently crushed. Different combinations of tailoring were tested: 1.2 mm Ductibor® (non-tailored), 1.6 mm Ductibor® (non-tailored), 1.2 mm Ductibor® - 1.2 mm Usibor® and 1.6 mm Ductibor® - 1.6 mm Usibor®. All four types of rails were subject to dynamic axial crush and also modelled in LS-DYNA. The models and experiments show a good match in deformation mode and force-displacement.
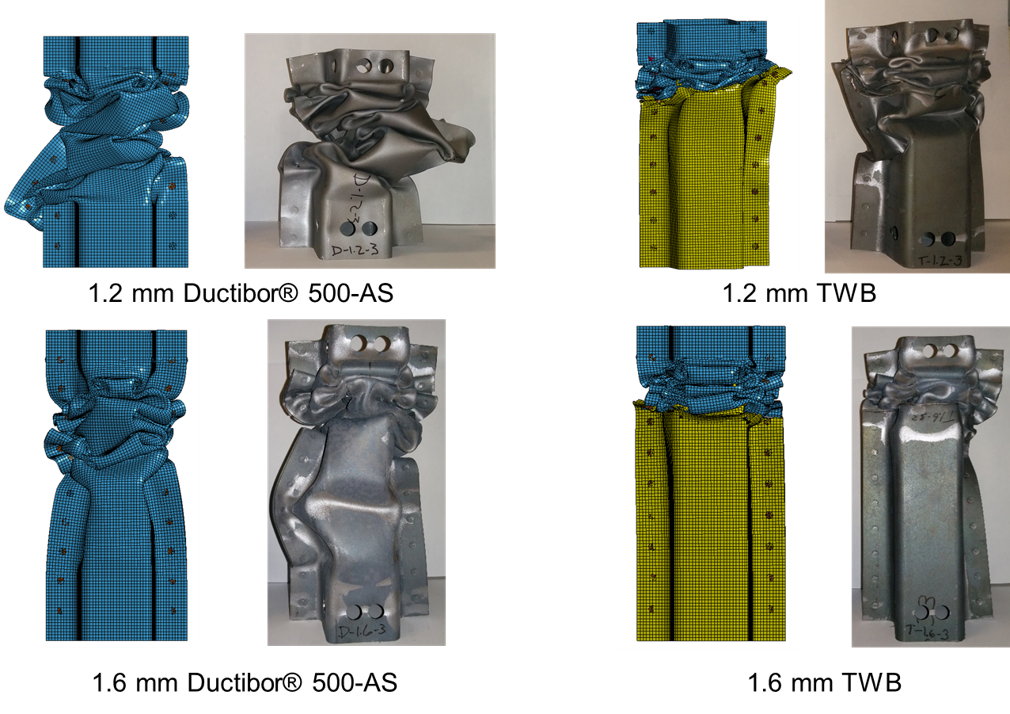
Finite element modelling alongside experimental results for the dynamic axial crush of tailor welded blanks
DP980
Top-hat shaped rails were brake-formed and spotwelded by Honda R&D Americas from three different batches of DP980 steel. Each material had different sheet thicknesses as well. All rails were subject to side impact and axial crush tests, dynamically and quasi-statically. There was a significant difference in deformation mode between the three batches.
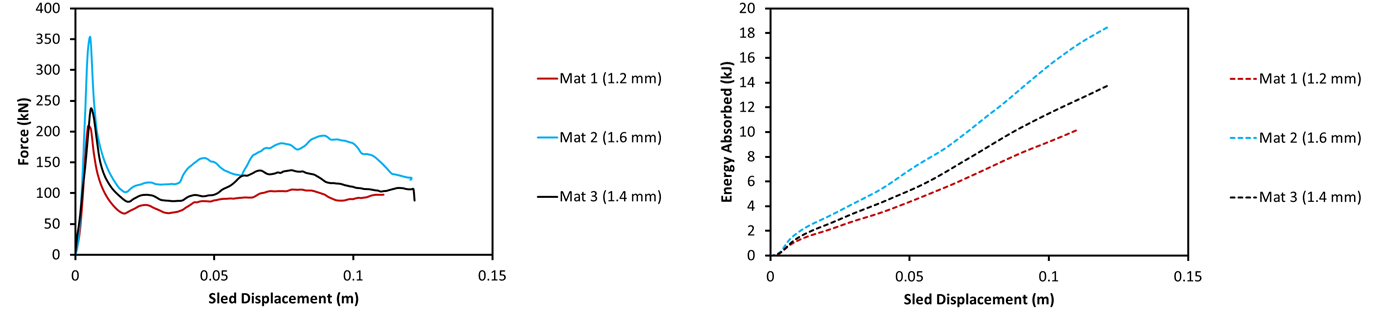
Quasi-static axial crush of DP980 rails
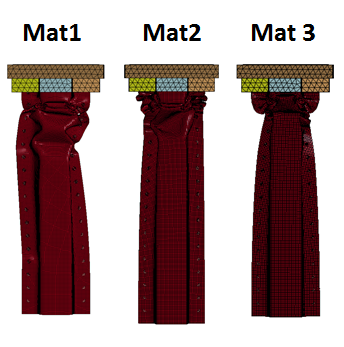
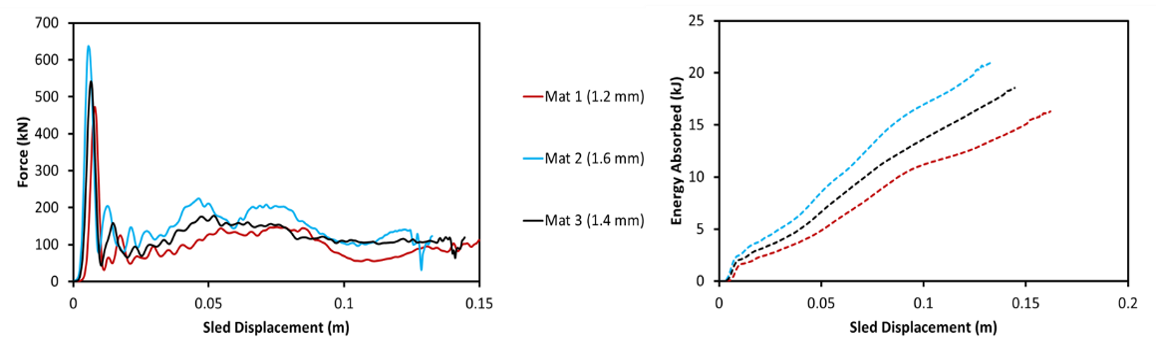
Dynamic axial crush of DP980 rails

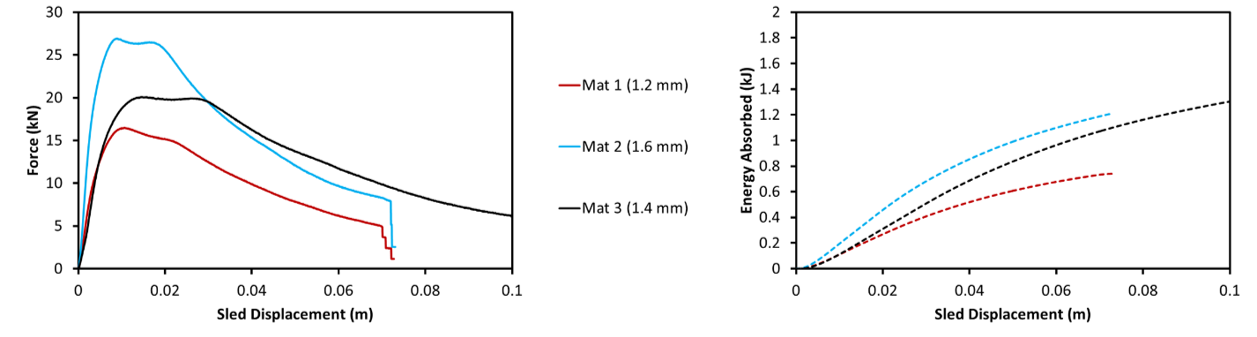
Dynamic side-impact of DP980 rails
6000 and 7000 series rails - Warm Formed and Die Quenched
Top hat-shaped rails made out of high strength aluminum alloys were warm formed and hot formed. Quasi-static and dynamic three-point bend tests were performed on warm formed AA6013-T6 and AA7075-T6 rails. Rails were formed and a backing plate, made of the same material as the rail, was riveted onto the rails.
Both materials were formed at 200 and 232°C isothermally and at 232°C non-isothermally. In general, rails formed under non-isothermal conditions exhibited poorer energy absorption response. In addition to warm forming, AA7075-T6 rails were also hot formed and subsequently subject to two heat treatments: a standard T6 treatment, and a custom treatment consisting of a temperature treatment replicating a paint bake cycle (177°C for 30 minutes). The custom temper was dubbed the T6IPB temper.
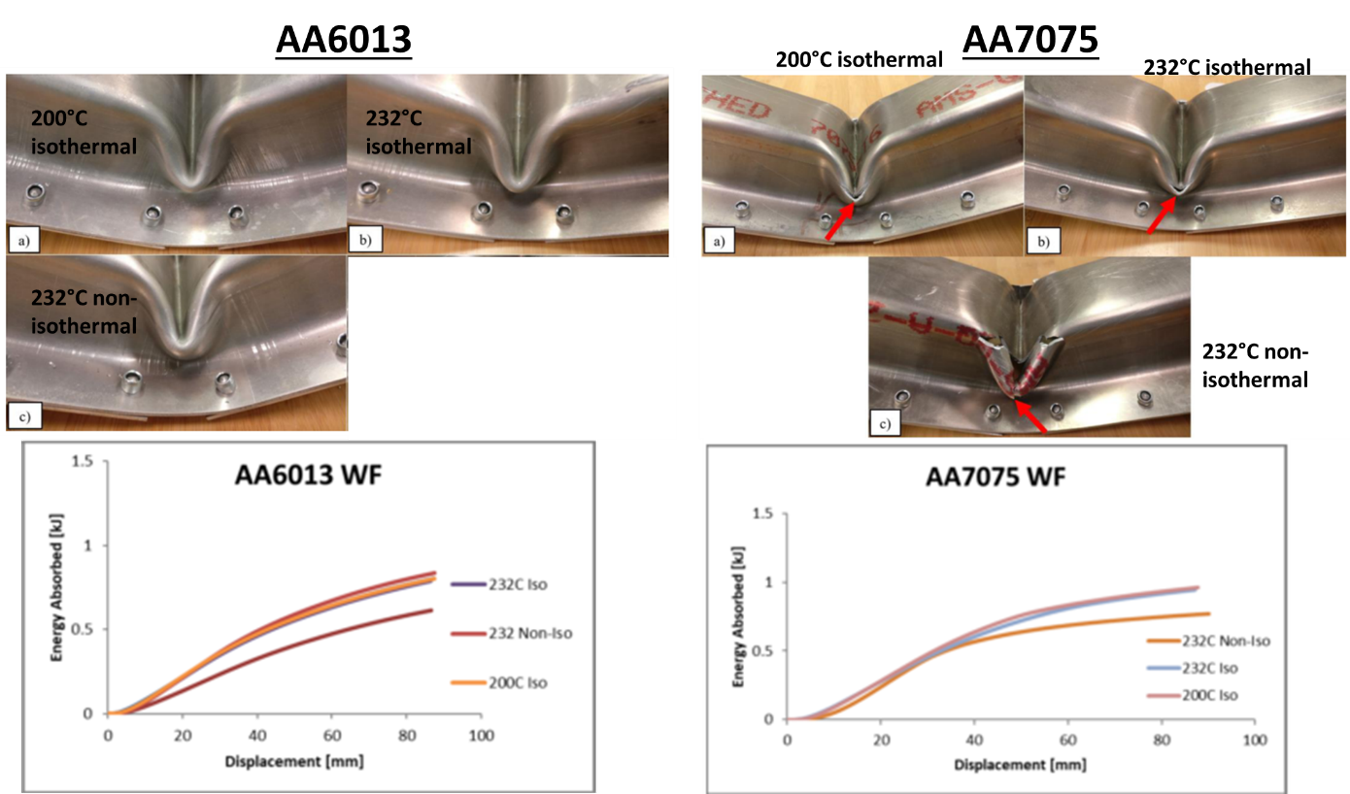
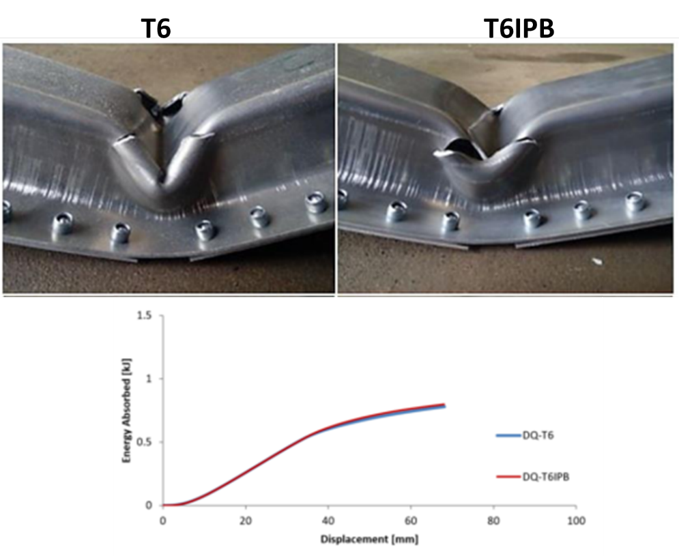
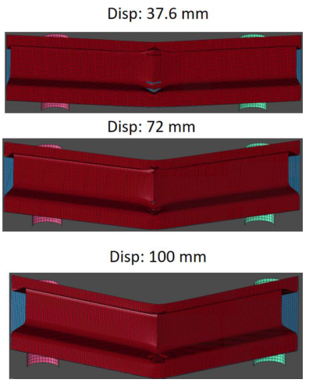
Quasi-static three-point bend results of hot formed AA7075 rails
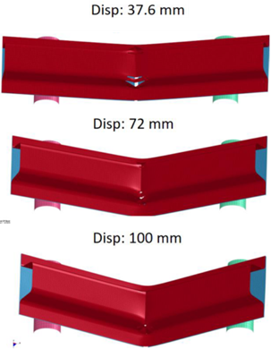
Dynamic three-point bend of hot formed AA7075-T6 rails