The uncertainty principle:
The position x and momentum p of a particle, e.g. an electron, cannot be simultaneously measured with arbitrary precision, i.e. the more accurately you measure the position, the less accurately you can measure the momentum. This principle is encoded in the following famous inequality:
(Δx)(Δp) ≤

where Δx (respectively Δp) is the uncertainty in the measurement of the position (respectively, the momentum) and h is the Planck constant.
Wave-particle duality:
In classical physics light was thought of as a wave phenomenon (for example it undergoes diffraction). However, the photoelectric effect can only be explained using quantum theory, with light being represented by photons (i.e. particles). In other words, light has a dual wave-particle nature.
On the other hand, electrons were thought of classically as (charged) particles. However, if a beam of electrons is passed through a thin metal film, an interference pattern is created, which can only be explained by assuming that the electrons have a wave-like nature. In other words, electrons also have a dual wave-particle nature. In quantum theory all particles are predicted to have a wave-like nature, leading to a universal wave-particle duality.
Anti-particles and anti-matter:
In 1927, the physicist Paul Dirac predicted the existence of a particle with the same mass as the electron but opposite (i.e. positive) charge. This particle, called the positron, was subsequently produced experimentally. The positron has the remarkable property that if it interacts with an electron both particles are annihilated and a pair of high energy photons is produced. The positron is called the antiparticle of the electron. Quantum theory predicts that almost every particle has its own antiparticle which will annihilate it. For example, the proton has an antiparticle called the anti-proton. In the past five years, physicists combined a positron with an anti-proton to form an anti-hydrogen atom, an example of anti-matter.
Quantum tunnelling:
A particle can violate the laws of classical mechanics by passing through a potential barrier whose energy is higher than the kinetic energy of the particle. In 1928, George Gamow used tunnelling to explain the radioactive decay of a nucleus via emission of an α-particle.
Superconductivity:
Superconductivity is the phenomenon whereby the electrical resistance of a material drops abruptly to zero when the material is cooled below its critical temperature. For example, mercury becomes a superconductor at a temperature of 4.2K (degrees Kelvin).
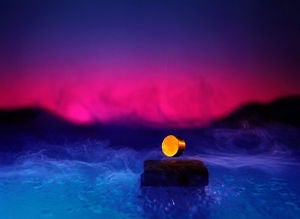
Quantum entanglement
Quantum entanglement is an intrinsically quantum mechanical phenomenon that is difficult to explain in classical terms. It refers to two quantum particles being linked together in a special way. Here is a quote from Paul Comstock, the author of "The Strange World of Quantum Entanglement" describing the phenomenon:
Entanglement is a strange feature of quantum physics, the science of the very small. It's possible to link together two quantum particles—photons of light or atoms, for example—in a special way that makes them effectively two parts of the same entity. You can then separate them as far as you like, and a change in one is instantly reflected in the other. This odd, faster than light link, is a fundamental aspect of quantum science.
(Reference, California Literary Review, <http://calitreview.com/2007/03/30/the-strange-world-of-quantum-entanglement/>)
The name "entanglement" was coined in the 1935 by Erwin Schrödinger, one of the founders of quantum mechanics, but the phenomenon was only demonstrated physically in the 1980's. It is currently the focus of much research because of the realization that entangled states are a physical resource with the potential to lead to dramatically new technology, for example, quantum cryptographic systems and quantum computers.