The Waterloo Institute for Nanotechnology (WIN) is comprized of many talented faculty members, students and researchers from various backgrounds of study. We wanted to showcase their incredible work through our Member Seminar Series! Each month a professor and 2 of their researchers will present their research to our community. This series is an opportunity for our WIN community to come together, learn about ongoing research and potentially foster new partnerships between students, faculty and labs.
"Performance and structural stability of cathodic electrocatalysts with complex nanoscale morphology"- Professor Anna Klinkova
Abstract
Increasing complexity of nanosynthesis has produced a variety of intricate nanoparticle shapes which have attracted significant attention for applications in electrocatalysis. These complex nanoelectrocatalysts not only introduce high surface areas and high energy active sites, but also improve electrocatalytic performance by altering electric field at the surface thereby concentrating reagents and accelerating electrocatalysis. However, long-term structural stability of complex nanoelectrocatalysts necessary for their industrial implementation often remains unknown and difficult to predict. This talk will focus on the structural stability of noble metal nanoparticles with star-, frame- and core-cage morphology in cathodic reactions (CO2 and water electrolysis). These examples collectively cover major structural nanoscale features including extrusions, holes, voids, and nanogaps. Based on the structural and surface analysis, density functional theory and finite element method calculations, uneven electric field and current density distributions in these morphologies influence metal mobility and cause structural degradation of certain features. Our results revealed that extrusions and holes facing the anode form electric field hot spots for electrolysis, that also facilitate surface reconstruction. The holes and voids perpendicular to the direction facing the anode form current crowding spots within the metal that facilitate electromigration via local Joule heating, while being blind spots for electrolysis due to low local electric field in these areas. Finally, structural features within nanocages are electrically shielded resulting in them being blind spots for both electrolysis and electromigration. This study provides guidelines for the structural design of electrocatalysts, highlighting that it should not only be dictated by the surface atomic organization and the generation of electric field hotspots, but also by current distribution within the electrode itself, to ensure high material performance and stability.
"The study of structural stability and catalytic activity of nanocatalysts: DFT approaches" - Feng Li
Abstract
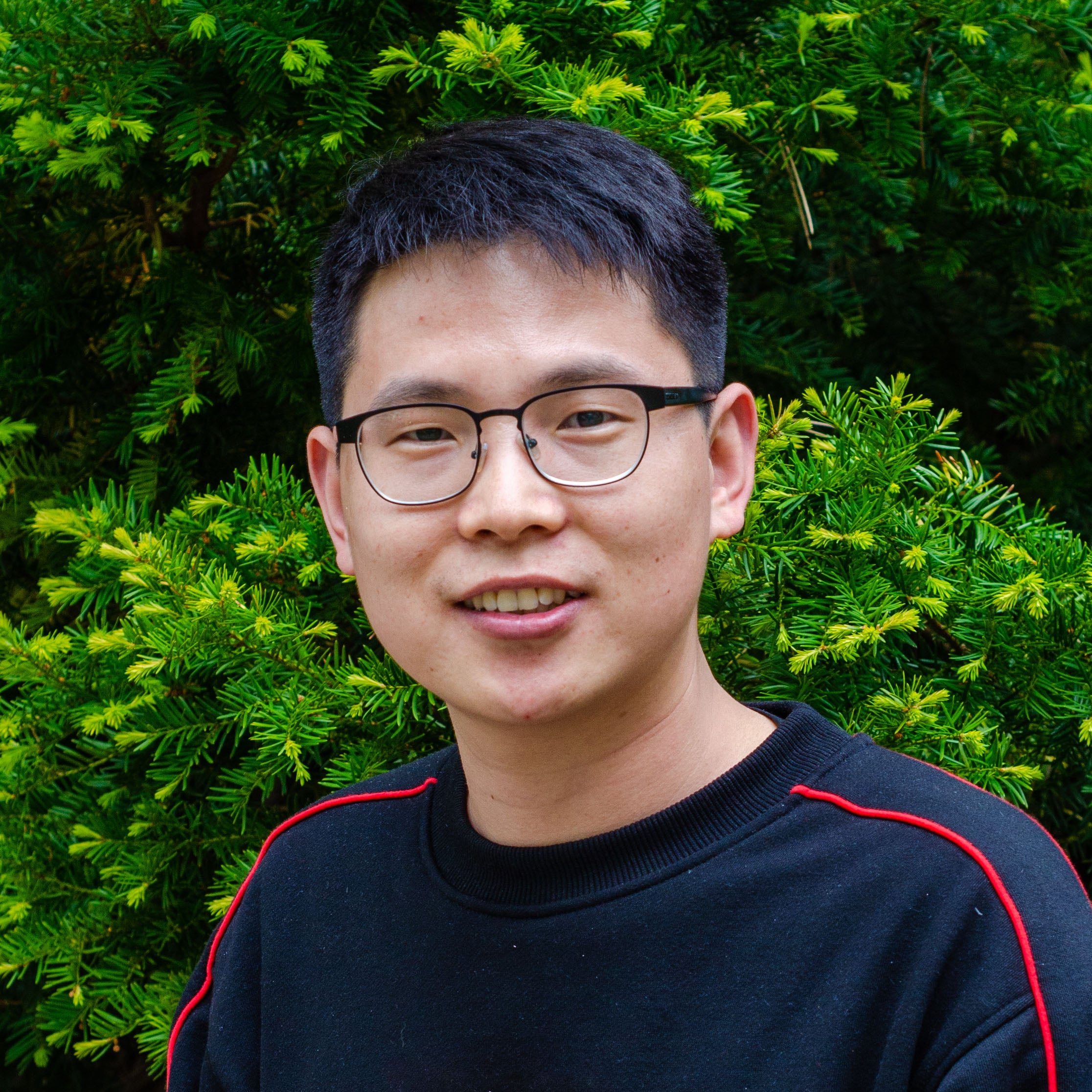
With rising global energy demand and environmental pollution, it is of urgent need to develop clean energy technologies such as catalytic and electrochemical hydrogen production and carbon dioxide electroreduction to fuels. Development of efficient catalysts, which are the heart of these technologies, requires detailed understanding of the nature, structural stability and activity of catalytic active sites. Density functional theory (DFT) is a powerful tool for exploring the structural stability and reaction mechanisms of catalysts at the atomic level. Obtained mechanistic information has a potential to predict catalytic properties of not yet explored catalysts, guiding the development of new nanomaterials for these applications.
In this talk, I will demonstrate three examples of using DFT calculations to explore structural stability and catalytic activity of nanocatalysts, specifically: (1) the influence of bound intermediates on the mobility of metal atoms at the catalyst surface related to its structural stability in water and carbon dioxide electroreduction; (2) the enhancement of catalytic performance of Pd nanocatalysts at CNT-Pd interface for formic acid dehydrogenation reaction; (3) the impact of potential-dependent adsorption of reaction intermediates on the outcome of electrocarboxylation reaction at Ag nanocatalysts. Computational hydrogen electrode, surface charging method, and Poisson-Boltzmann equation method were employed in these calculations.
"Synthetic strategies for shape-defined metal nanoparticles for catalytic applications" - Xenia Medvedeva
Abstract
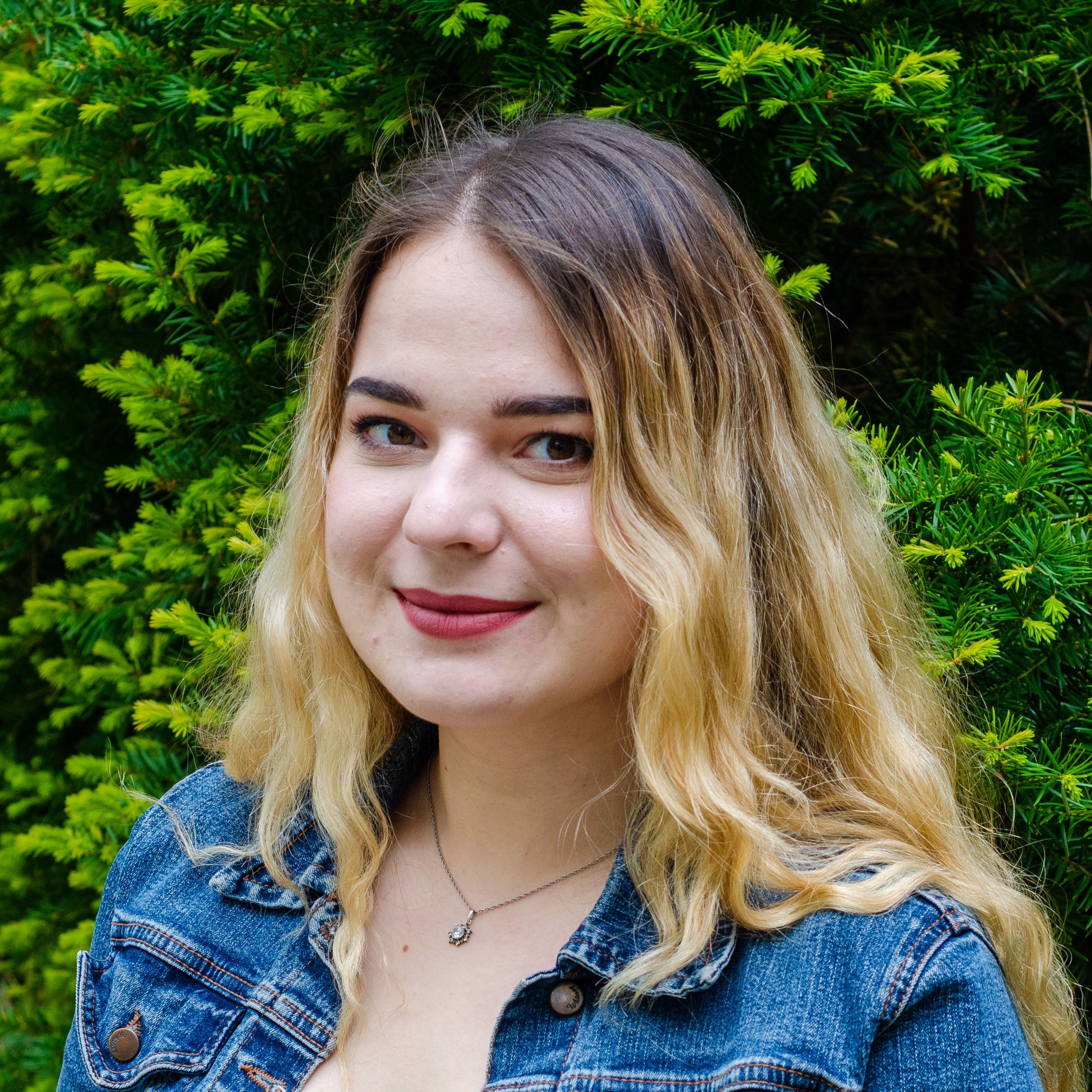
Metal nanoparticles entice attention due to their superior performance in various catalytic applications such as electrocatalysis, photocatalysis, CO oxidation, selective hydrogenation, and selective oxidation. Unlike bulk metals, nanoparticles have high surface-to-volume ratio, which is economically crucial for noble metal catalysis, and widely tunable properties due to their sensitivity to the size and shape variations. The change in a nanoparticle shape is connected to the difference in the atomic organization on the surface of a particle (in crystallographic facets, which have different surface energies) that affects its catalytic activity, selectivity, and stability. Thus, the ability to finely tune the shape of a nanomaterial by tweaking synthetic procedures is of high importance for the catalyst design.
Nanoparticles with simple geometric shapes, for instance cubes or dodecahedrons, expose specific low-index facets that exhibit facet-dependent catalytic activity, while nanoparticles with more complex morphology, for example hollow or with extrusions, have high-index facets that often act as extremely reactive catalytic sites due to the high density of steps and the presence of under-coordinated atoms with high surface energy. This talk will describe synthetic strategies for shape-defined metal nanoparticles with the following features: 1) specific low-index facets - via shape-directed growth; 2) branches - via the addition of an assisting metal; 3) cages and frames - via the removal of a core material from a core-shell structure; and 4) surface “nano-fingerprints” – via specific surface patterning.