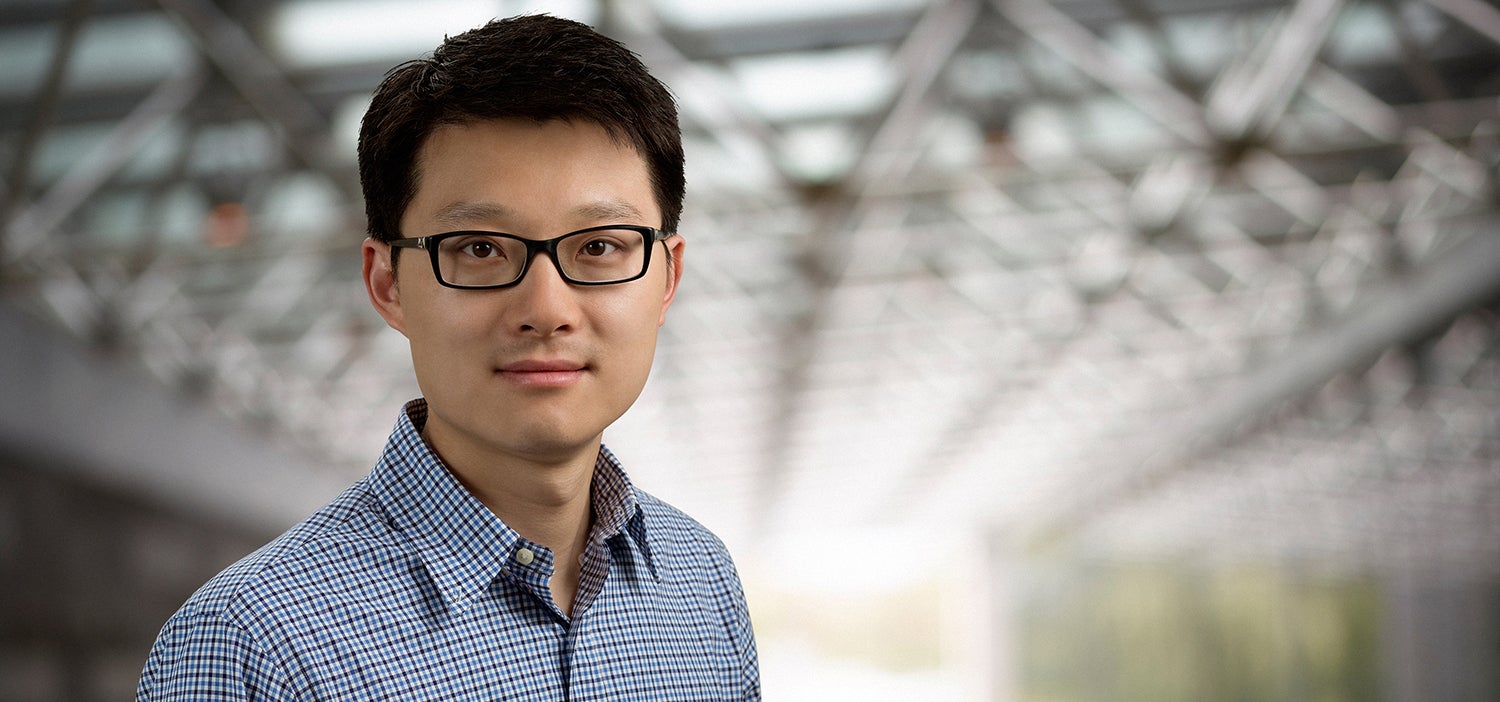
At the forefront of condensed matter physics, materials with novel quantum mechanical properties are being investigated for fundamental science and potential applications in quantum technology. Quantum chemist Dr. Adam Wei Tsen and his team are diving into this field with a particular focus on two-dimensional (2D) quantum materials.
The team is exploring how the magnetic properties of the layered material alpha ruthenium chloride (α-RuCl3) change in samples that approach the atomically thin 2D limit. At the atomic level, each electron has a quantity called spin, which gives rise to varying magnetic properties based on how the neighbouring spins interact. These interactions can also differ significantly between a single layer material and the bulk 3-dimensional sample of the same material consisting of many stacked layers. Tsen’s team is the first to study the magnetic properties of α-RuCl3 in its single layer form.
In its bulk form, α-RuCl3 hosts a unique interaction between neighbouring spins, called the Kitaev interaction, named after Russian-American physicist Alexei Kitaev. Kitaev’s work mathematically proved that when this is the only magnetic interaction in a 2D material with a honeycomb-shaped structure, the spins are entangled and exist in a liquid-like state, even at extremely low temperatures. If researchers can find a 2D material where the Kitaev interaction is more significant than all other spin interactions, it may lead to future applications in quantum information processing.
Unlike in theoretical predictions, in real materials the Kitaev interaction always competes with other ordinary spin interactions, and so careful tuning is required to engineer the magnetic properties. Tsen’s team studied how different sample thicknesses impact the magnetic properties of α-RuCl3.
“As α-RuCl3 has a layered honeycomb structure, we can isolate a single atomic layer of these materials with relative ease,” says Tsen, an Assistant Professor in the Department of Chemistry and at the Institute for Quantum Computing (IQC). “That allows us to study this quantum material in a form that is closer to the model system proposed by Kitaev.”
In these experiments, IQC researcher Dr. Bowen Yang, a postdoctoral fellow supervised by Tsen, used a nanoscale device geometry borrowed from spintronics (spin-based electronics), called the magnetic tunnel junction, which is used in today’s memory storage technology. In this device, thin layers of α-RuCl3 were isolated and sandwiched between two conducting materials. By examining how electrons tunneled through the insulating α-RuCl3, Yang was able to directly measure the material’s spin wave excitations down to the monolayer. The researchers found that, in contrast to the bulk crystal, the magnetic field needed to polarize the spins in the 2D sample was smaller in the direction perpendicular to the hexagonal layer structure than in plane with it.
The team’s collaborators from the University of Michigan then carefully examined the structure of monolayer α-RuCl3 using a technique called electron diffraction and found that it exhibited several distortions relative to the bulk crystal. Theorists from Goethe University Frankfurt and Wake Forest University then calculated the magnetic interactions from this distorted structure. They successfully explained the change in the effect of the magnetic field, and further discovered that the Kitaev interaction was substantially increased. Collectively, these findings highlight the importance of dimensionality in tuning the properties of magnetic materials and demonstrate that monolayer α-RuCl3 may be more relevant for quantum computing applications than its bulk counterpart.
At the same time, a perpendicular magnetic field has been theoretically predicted to induce spin liquid phases even when the system was not a spin liquid to begin with. “What’s known about the bulk crystal is that you have to apply an extremely large magnetic field of about 35 Tesla to drive it out of its ground state, which is largely inaccessible by most groups,” says Tsen. “But it only takes about 6 Tesla, a much smaller magnetic field, to break this ground state for a single layer, which could be very promising for the future”.
The paper “Magnetic anisotropy reversal driven by structural symmetry-breaking in monolayer α-RuCl3” was published last week in Nature Materials. Yang recently won the IQC achievement award for this work. The research was funded in part by the US Army Research Office as well as Transformative Quantum Technologies at the University of Waterloo.