Why do our veins look blue when blood is red? This is a seemingly elementary science trivia question, and certainly not one that computer science researchers would be expected to be interested in.
But Spencer Van Leeuwen, who recently completed his Master of Mathematics in Computer Science at the University of Waterloo, and his supervisor Professor Gladimir Baranoski, who heads the Natural Phenomena Simulation Group in the David R. Cheriton School of Computer Science, have recently shed light on exactly why veins look blue.
The question is much more complex than it seems, and by tackling it using computer models that simulate organic structures, Van Leeuwen and Baranoski have elucidated the details how light interacts with the cellular and subcellular parts of the skin and blood to clarify how veins get their bluish appearance.
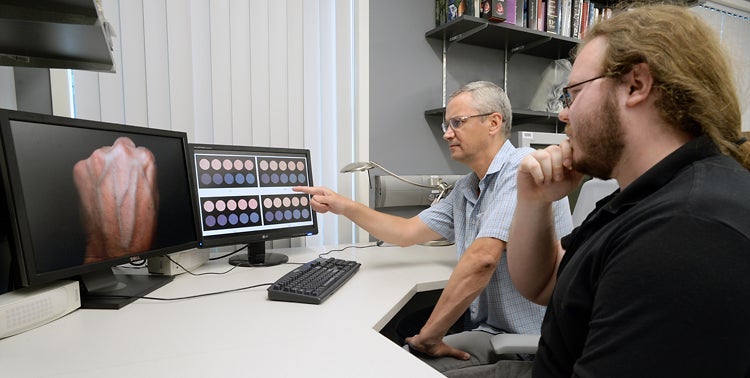
Professor Gladimir
Baranoski
(left)
and
his
master's
student Spencer Van
Leeuwen
examine
computer-generated
swatches
depicting
the
effect
of
Rayleigh
scattering
in
the
papillary
dermis
on
the
appearance
of
a skin
specimen
without
(pink
circles)
and
with
(blue
circles)
a
subcutaneous
vein.
The
monitor
on
the
left
shows
the
typical
distinct
contrast
of
skin
appearance
caused
by
subcutaneous
veins.
Ultimately, the work that Van Leeuwen and Baranoski have done in clarifying why veins look blue will add to the knowledge base that is needed for technologies that are capable of non-invasive diagnostic testing. There are some instruments today such as devices that measure the amount of oxygen in the blood without pricking the skin, and people have long dreamed of having a Star Trek-like tricorder that can scan for medical problems in a less invasive way. But for those types of instruments to work well, the fundamental data is important to ensure that the results are accurate.
“What we are doing is contributing to the understanding of what is happening inside our tissues,” Baranoski says.
Simulating how materials absorb and propagate light is known as “appearance modelling,” and can be applied to anything from a table, to a flower or to the human skin and blood, Baranoski says. It has long been an aspect of computer graphics used in computer games, but now it has the potential for a much wider range of applications, far beyond entertainment, he adds. “Computer science has to expand. It has strong potential to help in other areas.”
Decades
ago,
researchers
realized
that
the
bluish
colour
of
veins
might
have
something
to
do
with
the
scattering
of
light,
particularly
a
type
of
scattering
known
as
Rayleigh
scattering,
which
is
what
causes
the
sky
to
look
blue.
Since
blue
light
has
shorter,
smaller
wavelengths,
it
is
more
likely
to
be
scattered
when
it
bumps
into
molecules
of
the
atmosphere,
giving
the
sky
its
blue
appearance.
It
was
strongly
suspected
that
that
Rayleigh
scattering,
named
after
British
physicist
John
William
Strutt,
also
known
as
Lord
Rayleigh,
was
also
involved
in
the
bluish
appearance
of
veins.
But
the
question
of
exactly
what
is
happening
at
the
cellular
and
subcellular
level
to
cause
the
scattering
eluded
researchers.
It
wasn’t
until
the
mid-1990s
that
researchers
started
to
look
more
closely
at
how
the
skin
and
vessels
might
contribute
to
the
scattering
of
the
light.
They
found
that
blue
light
does
not
penetrate
as
deeply
into
the
skin
as
red
and
green
light,
which
is
more
likely
to
be
absorbed
within
the
blood.
The
blue
light
therefore
has
more
of
a
tendency
to
be
scattered
away
from
the
blood,
giving
veins
the
bluish
appearance.
This,
along
with
the
way
humans
perceive
colour,
helped
to
explain
the
blue
appearance
of
veins.
But
even
then,
the
researchers
were
not
able
to
pinpoint
exactly
what
structures
in
the
skin
were
scattering
the
light
and
get
details
of
how
the
scattering
worked.
This
was
where
Van
Leeuwen,
working
in
Baranoski’s
group,
made
a
big
contribution.
The research involved using two sophisticated modelling tools that Baranoski and his students have developed at Waterloo over the years. One is called HyLIoS (Hyperspectral Light Impingement on Skin) that simulates the interaction of light with skin. The other model, that Van Leeuwen helped improve, is called CLBlood (Cell-Based Light Interaction Model for Human Blood), and it simulates how light is absorbed and propagated by blood flowing in the veins. Both employ computational algorithms known as the Monte Carlo methods, that rely on repeated random sampling to obtain results.
The tools developed at Waterloo are powerful enough to account for many more details in the properties of the skin and blood, such as the way that the distribution of pigment-containing structures known as melanosomes help to scatter the light in the skin and blood, and the location and orientation of cell structures.
Using these tools, it was possible to plug in the parameters for how different types of light coming in at different angles and and simulate how small tissues structures, known as collagen fibrils, scatter light and ultimately affect the colour of the skin and veins. The resulting colour variations are then reproduced using skin swatches generated using techniques employed in computer graphics.
He was then able to validate these models against measurements cited in research literature that describes the physical properties of the skin, vein and blood structures. “If, in the literature, it says that if you reduce the amount of melanin, the skin will appear lighter, we can reduce the melanin and see if the skin appears lighter,” Van Leeuwen says. He was also able to compare the results against how humans see the colours of the skin and veins. “We did every type of validation that we were capable of, given the measurements in the literature.”
Baranoski says using computers, is possible to turn the Rayleigh scattering on or off, for example, and see how the colour changes. It also becomes possible to differentiate between one type of light scattering and another and see the effects.
“These models are tools for us, and you can imagine them as a kind of microscope,” Baranoski says.
Their paper, Elucidating the Contribution of Rayleigh Scattering to the Bluish Appearance of Veins, recently appeared in the Journal of Biomedical Optics.